Oxygen Sensing by T Cells Establishes an Immunologically Tolerant Metastatic Niche
- PMID: 27565342
- PMCID: PMC5548538
- DOI: 10.1016/j.cell.2016.07.032
Oxygen Sensing by T Cells Establishes an Immunologically Tolerant Metastatic Niche
Abstract
Cancer cells must evade immune responses at distant sites to establish metastases. The lung is a frequent site for metastasis. We hypothesized that lung-specific immunoregulatory mechanisms create an immunologically permissive environment for tumor colonization. We found that T-cell-intrinsic expression of the oxygen-sensing prolyl-hydroxylase (PHD) proteins is required to maintain local tolerance against innocuous antigens in the lung but powerfully licenses colonization by circulating tumor cells. PHD proteins limit pulmonary type helper (Th)-1 responses, promote CD4(+)-regulatory T (Treg) cell induction, and restrain CD8(+) T cell effector function. Tumor colonization is accompanied by PHD-protein-dependent induction of pulmonary Treg cells and suppression of IFN-γ-dependent tumor clearance. T-cell-intrinsic deletion or pharmacological inhibition of PHD proteins limits tumor colonization of the lung and improves the efficacy of adoptive cell transfer immunotherapy. Collectively, PHD proteins function in T cells to coordinate distinct immunoregulatory programs within the lung that are permissive to cancer metastasis. PAPERCLIP.
Published by Elsevier Inc.
Figures
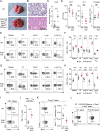
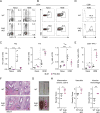
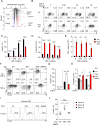
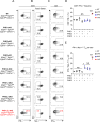
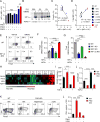
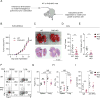
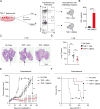
Similar articles
-
Tc1 and Tc2 effector cell therapy elicit long-term tumor immunity by contrasting mechanisms that result in complementary endogenous type 1 antitumor responses.J Immunol. 2004 Feb 1;172(3):1380-90. doi: 10.4049/jimmunol.172.3.1380. J Immunol. 2004. PMID: 14734713
-
Role of effector cell-derived IL-4, IL-5, and perforin in early and late stages of type 2 CD8 effector cell-mediated tumor rejection.J Immunol. 2001 Jul 1;167(1):424-34. doi: 10.4049/jimmunol.167.1.424. J Immunol. 2001. PMID: 11418679
-
IL-4-transduced tumor cell vaccine induces immunoregulatory type 2 CD8 T lymphocytes that cure lung metastases upon adoptive transfer.J Immunol. 1999 Aug 15;163(4):1923-8. J Immunol. 1999. PMID: 10438927
-
Depletion of CD4(+)CD25(high) regulatory T cells from tumor infiltrating lymphocytes predominantly induces Th1 type immune response in vivo which inhibits tumor growth in adoptive immunotherapy.Cancer Biol Ther. 2009 Jan;8(1):66-72. doi: 10.4161/cbt.8.1.7131. Epub 2009 Jan 4. Cancer Biol Ther. 2009. PMID: 19029829
-
Type 1 and type 2 CD8+ effector T cell subpopulations promote long-term tumor immunity and protection to progressively growing tumor.J Immunol. 2000 Jan 15;164(2):916-25. doi: 10.4049/jimmunol.164.2.916. J Immunol. 2000. PMID: 10623840
Cited by
-
The oxygen sensor prolyl hydroxylase domain 2 regulates the in vivo suppressive capacity of regulatory T cells.Elife. 2022 Feb 22;11:e70555. doi: 10.7554/eLife.70555. Elife. 2022. PMID: 35192456 Free PMC article.
-
Changes in Pulmonary Microenvironment Aids Lung Metastasis of Breast Cancer.Front Oncol. 2022 May 26;12:860932. doi: 10.3389/fonc.2022.860932. eCollection 2022. Front Oncol. 2022. PMID: 35719975 Free PMC article. Review.
-
Mitochondria: the gatekeepers between metabolism and immunity.Front Immunol. 2024 Feb 23;15:1334006. doi: 10.3389/fimmu.2024.1334006. eCollection 2024. Front Immunol. 2024. PMID: 38464536 Free PMC article. Review.
-
The therapeutic implications of immunosuppressive tumor aerobic glycolysis.Cell Mol Immunol. 2022 Jan;19(1):46-58. doi: 10.1038/s41423-021-00727-3. Epub 2021 Jul 8. Cell Mol Immunol. 2022. PMID: 34239083 Free PMC article. Review.
-
Influenza-Specific Lung-Resident Memory CD8+ T Cells.Cold Spring Harb Perspect Biol. 2021 Feb 1;13(2):a037978. doi: 10.1101/cshperspect.a037978. Cold Spring Harb Perspect Biol. 2021. PMID: 33288540 Free PMC article. Review.
References
Publication types
MeSH terms
Substances
Grants and funding
- BBS/E/B/000C0407/BB_/Biotechnology and Biological Sciences Research Council/United Kingdom
- R01 AI096852/AI/NIAID NIH HHS/United States
- BB/N007794/1/BB_/Biotechnology and Biological Sciences Research Council/United Kingdom
- Z01 BC010763-03/Intramural NIH HHS/United States
- T32 GM007240/GM/NIGMS NIH HHS/United States
LinkOut - more resources
Full Text Sources
Other Literature Sources
Medical
Molecular Biology Databases
Research Materials