Kank2 activates talin, reduces force transduction across integrins and induces central adhesion formation
- PMID: 27548916
- PMCID: PMC6053543
- DOI: 10.1038/ncb3402
Kank2 activates talin, reduces force transduction across integrins and induces central adhesion formation
Abstract
Integrin-based adhesions play critical roles in cell migration. Talin activates integrins and flexibly connects integrins to the actomyosin cytoskeleton, thereby serving as a 'molecular clutch' that transmits forces to the extracellular matrix to drive cell migration. Here we identify the evolutionarily conserved Kank protein family as novel components of focal adhesions (FAs). Kank proteins accumulate at the lateral border of FAs, which we term the FA belt, and in central sliding adhesions, where they directly bind the talin rod domain through the Kank amino-terminal (KN) motif and induce talin and integrin activation. In addition, Kank proteins diminish the talin-actomyosin linkage, which curbs force transmission across integrins, leading to reduced integrin-ligand bond strength, slippage between integrin and ligand, central adhesion formation and sliding, and reduced cell migration speed. Our data identify Kank proteins as talin activators that decrease the grip between the integrin-talin complex and actomyosin to regulate cell migration velocity.
Conflict of interest statement
The authors declare no competing financial interests.
Figures
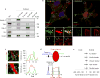
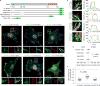
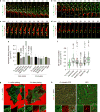
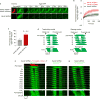
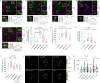
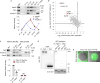
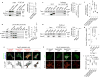
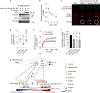
Similar articles
-
Vinculin regulates the recruitment and release of core focal adhesion proteins in a force-dependent manner.Curr Biol. 2013 Feb 18;23(4):271-81. doi: 10.1016/j.cub.2013.01.009. Epub 2013 Jan 31. Curr Biol. 2013. PMID: 23375895 Free PMC article.
-
Vinculin controls focal adhesion formation by direct interactions with talin and actin.J Cell Biol. 2007 Dec 3;179(5):1043-57. doi: 10.1083/jcb.200703036. J Cell Biol. 2007. PMID: 18056416 Free PMC article.
-
Vinculin controls talin engagement with the actomyosin machinery.Nat Commun. 2015 Dec 4;6:10038. doi: 10.1038/ncomms10038. Nat Commun. 2015. PMID: 26634421 Free PMC article.
-
Molecular mechanisms underlying the force-dependent regulation of actin-to-ECM linkage at the focal adhesions.Prog Mol Biol Transl Sci. 2014;126:135-54. doi: 10.1016/B978-0-12-394624-9.00006-3. Prog Mol Biol Transl Sci. 2014. PMID: 25081617 Review.
-
New insights into vinculin function and regulation.Int Rev Cell Mol Biol. 2011;287:191-231. doi: 10.1016/B978-0-12-386043-9.00005-0. Int Rev Cell Mol Biol. 2011. PMID: 21414589 Free PMC article. Review.
Cited by
-
Critical role of lipid membranes in polarization and migration of cells: a biophysical view.Biophys Rev. 2021 Jan 11;13(1):123-138. doi: 10.1007/s12551-021-00781-1. eCollection 2021 Feb. Biophys Rev. 2021. PMID: 33747247 Free PMC article. Review.
-
The role and regulation of integrins in cell migration and invasion.Nat Rev Mol Cell Biol. 2024 Sep 30. doi: 10.1038/s41580-024-00777-1. Online ahead of print. Nat Rev Mol Cell Biol. 2024. PMID: 39349749 Review.
-
Superresolution architecture of cornerstone focal adhesions in human pluripotent stem cells.Nat Commun. 2019 Oct 18;10(1):4756. doi: 10.1038/s41467-019-12611-w. Nat Commun. 2019. PMID: 31628312 Free PMC article.
-
A Layered View on Focal Adhesions.Biology (Basel). 2021 Nov 16;10(11):1189. doi: 10.3390/biology10111189. Biology (Basel). 2021. PMID: 34827182 Free PMC article. Review.
-
Key Roles of RGD-Recognizing Integrins During Cardiac Development, on Cardiac Cells, and After Myocardial Infarction.J Cardiovasc Transl Res. 2022 Feb;15(1):179-203. doi: 10.1007/s12265-021-10154-4. Epub 2021 Aug 3. J Cardiovasc Transl Res. 2022. PMID: 34342855 Review.
References
-
- Giannone G, Mege RM, Thoumine O. Multi-level molecular clutches in motile cell processes. Trends Cell Biol. 2009;19:475–486. - PubMed
MeSH terms
Substances
Grants and funding
LinkOut - more resources
Full Text Sources
Other Literature Sources
Molecular Biology Databases
Research Materials
Miscellaneous