Link of a ubiquitous human coronavirus to dromedary camels
- PMID: 27528677
- PMCID: PMC5024591
- DOI: 10.1073/pnas.1604472113
Link of a ubiquitous human coronavirus to dromedary camels
Abstract
The four human coronaviruses (HCoVs) are globally endemic respiratory pathogens. The Middle East respiratory syndrome (MERS) coronavirus (CoV) is an emerging CoV with a known zoonotic source in dromedary camels. Little is known about the origins of endemic HCoVs. Studying these viruses' evolutionary history could provide important insight into CoV emergence. In tests of MERS-CoV-infected dromedaries, we found viruses related to an HCoV, known as HCoV-229E, in 5.6% of 1,033 animals. Human- and dromedary-derived viruses are each monophyletic, suggesting ecological isolation. One gene of dromedary viruses exists in two versions in camels, full length and deleted, whereas only the deleted version exists in humans. The deletion increased in size over a succession starting from camelid viruses via old human viruses to contemporary human viruses. Live isolates of dromedary 229E viruses were obtained and studied to assess human infection risks. The viruses used the human entry receptor aminopeptidase N and replicated in human hepatoma cells, suggesting a principal ability to cause human infections. However, inefficient replication in several mucosa-derived cell lines and airway epithelial cultures suggested lack of adaptation to the human host. Dromedary viruses were as sensitive to the human type I interferon response as HCoV-229E. Antibodies in human sera neutralized dromedary-derived viruses, suggesting population immunity against dromedary viruses. Although no current epidemic risk seems to emanate from these viruses, evolutionary inference suggests that the endemic human virus HCoV-229E may constitute a descendant of camelid-associated viruses. HCoV-229E evolution provides a scenario for MERS-CoV emergence.
Keywords: coronavirus; ecology; evolution; livestock; zoonotic diseases.
Conflict of interest statement
The authors declare no conflict of interest.
Figures
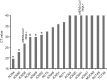
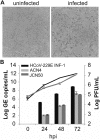
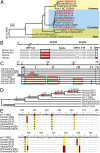
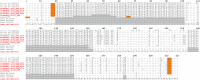

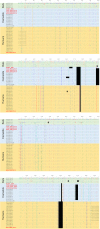
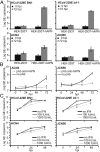
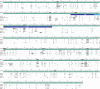
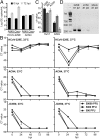
Similar articles
-
Co-circulation of three camel coronavirus species and recombination of MERS-CoVs in Saudi Arabia.Science. 2016 Jan 1;351(6268):81-4. doi: 10.1126/science.aac8608. Epub 2015 Dec 17. Science. 2016. PMID: 26678874
-
Hosts and Sources of Endemic Human Coronaviruses.Adv Virus Res. 2018;100:163-188. doi: 10.1016/bs.aivir.2018.01.001. Epub 2018 Feb 16. Adv Virus Res. 2018. PMID: 29551135 Free PMC article. Review.
-
Tropism and replication of Middle East respiratory syndrome coronavirus from dromedary camels in the human respiratory tract: an in-vitro and ex-vivo study.Lancet Respir Med. 2014 Oct;2(10):813-22. doi: 10.1016/S2213-2600(14)70158-4. Epub 2014 Aug 28. Lancet Respir Med. 2014. PMID: 25174549 Free PMC article.
-
Middle East respiratory syndrome coronavirus quasispecies that include homologues of human isolates revealed through whole-genome analysis and virus cultured from dromedary camels in Saudi Arabia.mBio. 2014 Apr 29;5(3):e01146-14. doi: 10.1128/mBio.01146-14. mBio. 2014. PMID: 24781747 Free PMC article.
-
Middle East Respiratory Syndrome Coronavirus (MERS-CoV) origin and animal reservoir.Virol J. 2016 Jun 3;13:87. doi: 10.1186/s12985-016-0544-0. Virol J. 2016. PMID: 27255185 Free PMC article. Review.
Cited by
-
Disparate temperature-dependent virus-host dynamics for SARS-CoV-2 and SARS-CoV in the human respiratory epithelium.PLoS Biol. 2021 Mar 29;19(3):e3001158. doi: 10.1371/journal.pbio.3001158. eCollection 2021 Mar. PLoS Biol. 2021. PMID: 33780434 Free PMC article.
-
Middle East Respiratory Coronavirus Accessory Protein 4a Inhibits PKR-Mediated Antiviral Stress Responses.PLoS Pathog. 2016 Oct 26;12(10):e1005982. doi: 10.1371/journal.ppat.1005982. eCollection 2016 Oct. PLoS Pathog. 2016. PMID: 27783669 Free PMC article.
-
Origins and Evolution of Seasonal Human Coronaviruses.Viruses. 2022 Jul 15;14(7):1551. doi: 10.3390/v14071551. Viruses. 2022. PMID: 35891531 Free PMC article.
-
Comparative Pathogenesis of Bovine and Porcine Respiratory Coronaviruses in the Animal Host Species and SARS-CoV-2 in Humans.J Clin Microbiol. 2020 Jul 23;58(8):e01355-20. doi: 10.1128/JCM.01355-20. Print 2020 Jul 23. J Clin Microbiol. 2020. PMID: 32522830 Free PMC article. Review.
-
Evolutionary Origins of Enteric Hepatitis Viruses.Cold Spring Harb Perspect Med. 2018 Dec 3;8(12):a031690. doi: 10.1101/cshperspect.a031690. Cold Spring Harb Perspect Med. 2018. PMID: 29610146 Free PMC article. Review.
References
Publication types
MeSH terms
Associated data
- Actions
- Actions
LinkOut - more resources
Full Text Sources
Other Literature Sources