Nucleolin-Mediated RNA Localization Regulates Neuron Growth and Cycling Cell Size
- PMID: 27477284
- PMCID: PMC4978702
- DOI: 10.1016/j.celrep.2016.07.005
Nucleolin-Mediated RNA Localization Regulates Neuron Growth and Cycling Cell Size
Abstract
How can cells sense their own size to coordinate biosynthesis and metabolism with their growth needs? We recently proposed a motor-dependent bidirectional transport mechanism for axon length and cell size sensing, but the nature of the motor-transported size signals remained elusive. Here, we show that motor-dependent mRNA localization regulates neuronal growth and cycling cell size. We found that the RNA-binding protein nucleolin is associated with importin β1 mRNA in axons. Perturbation of nucleolin association with kinesins reduces its levels in axons, with a concomitant reduction in axonal importin β1 mRNA and protein levels. Strikingly, subcellular sequestration of nucleolin or importin β1 enhances axonal growth and causes a subcellular shift in protein synthesis. Similar findings were obtained in fibroblasts. Thus, subcellular mRNA localization regulates size and growth in both neurons and cycling cells.
Copyright © 2016 The Author(s). Published by Elsevier Inc. All rights reserved.
Figures
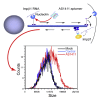
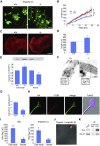
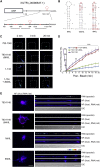
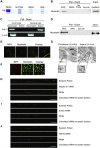
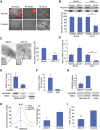
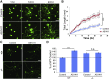
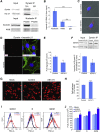
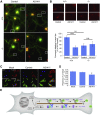
Similar articles
-
The glycine arginine-rich domain of the RNA-binding protein nucleolin regulates its subcellular localization.EMBO J. 2021 Oct 18;40(20):e107158. doi: 10.15252/embj.2020107158. Epub 2021 Sep 13. EMBO J. 2021. PMID: 34515347 Free PMC article.
-
Locally translated mTOR controls axonal local translation in nerve injury.Science. 2018 Mar 23;359(6382):1416-1421. doi: 10.1126/science.aan1053. Science. 2018. PMID: 29567716 Free PMC article.
-
Dynamics of survival of motor neuron (SMN) protein interaction with the mRNA-binding protein IMP1 facilitates its trafficking into motor neuron axons.Dev Neurobiol. 2014 Mar;74(3):319-332. doi: 10.1002/dneu.22111. Epub 2013 Oct 4. Dev Neurobiol. 2014. PMID: 23897586 Free PMC article.
-
Molecular mechanisms behind mRNA localization in axons.Open Biol. 2020 Sep;10(9):200177. doi: 10.1098/rsob.200177. Epub 2020 Sep 23. Open Biol. 2020. PMID: 32961072 Free PMC article. Review.
-
Molecular control of local translation in axon development and maintenance.Curr Opin Neurobiol. 2018 Aug;51:86-94. doi: 10.1016/j.conb.2018.02.025. Epub 2018 Mar 14. Curr Opin Neurobiol. 2018. PMID: 29549711 Review.
Cited by
-
Nucleolin acute degradation reveals novel functions in cell cycle progression and division in TNBC.bioRxiv [Preprint]. 2024 Jun 22:2024.06.17.599429. doi: 10.1101/2024.06.17.599429. bioRxiv. 2024. PMID: 38948867 Free PMC article. Preprint.
-
Riding the rails - different modes for RNA complex transport in axons.Neural Regen Res. 2022 Dec;17(12):2664-2665. doi: 10.4103/1673-5374.339478. Neural Regen Res. 2022. PMID: 35662201 Free PMC article. No abstract available.
-
Axonal localization of neuritin/CPG15 mRNA is limited by competition for HuD binding.J Cell Sci. 2017 Nov 1;130(21):3650-3662. doi: 10.1242/jcs.201244. Epub 2017 Sep 4. J Cell Sci. 2017. PMID: 28871047 Free PMC article.
-
COPI coatomer subunit α-COP interacts with the RNA binding protein Nucleolin via a C-terminal dilysine motif.Hum Mol Genet. 2023 Nov 17;32(23):3263-3275. doi: 10.1093/hmg/ddad140. Hum Mol Genet. 2023. PMID: 37658769 Free PMC article.
-
The functional organization of axonal mRNA transport and translation.Nat Rev Neurosci. 2021 Feb;22(2):77-91. doi: 10.1038/s41583-020-00407-7. Epub 2020 Dec 7. Nat Rev Neurosci. 2021. PMID: 33288912 Free PMC article. Review.
References
MeSH terms
Substances
Grants and funding
LinkOut - more resources
Full Text Sources
Other Literature Sources
Molecular Biology Databases