Bacterial Strategies to Maintain Zinc Metallostasis at the Host-Pathogen Interface
- PMID: 27462080
- PMCID: PMC5076499
- DOI: 10.1074/jbc.R116.742023
Bacterial Strategies to Maintain Zinc Metallostasis at the Host-Pathogen Interface
Abstract
Among the biologically required first row, late d-block metals from MnII to ZnII, the catalytic and structural reach of ZnII ensures that this essential micronutrient touches nearly every major metabolic process or pathway in the cell. Zn is also toxic in excess, primarily because it is a highly competitive divalent metal and will displace more weakly bound transition metals in the active sites of metalloenzymes if left unregulated. The vertebrate innate immune system uses several strategies to exploit this "Achilles heel" of microbial physiology, but bacterial evolution has responded in kind. This review highlights recent insights into transcriptional, transport, and trafficking mechanisms that pathogens use to "win the fight" over zinc and thrive in an otherwise hostile environment.
Keywords: ABC transporter; allostery; antibiotics; bacteria; host-pathogen interaction; metal transporter; metallochaperone; metallophores; metalloprotein; metalloregulatory protein; metallostasis; zinc; zinc homeostasis.
© 2016 by The American Society for Biochemistry and Molecular Biology, Inc.
Figures
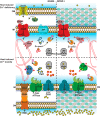
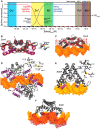
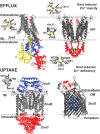
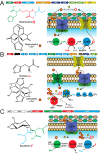
Similar articles
-
Metallochaperones and metalloregulation in bacteria.Essays Biochem. 2017 May 9;61(2):177-200. doi: 10.1042/EBC20160076. Print 2017 May 9. Essays Biochem. 2017. PMID: 28487396 Free PMC article. Review.
-
Copper transport and trafficking at the host-bacterial pathogen interface.Acc Chem Res. 2014 Dec 16;47(12):3605-13. doi: 10.1021/ar500300n. Epub 2014 Oct 13. Acc Chem Res. 2014. PMID: 25310275 Free PMC article.
-
Multi-metal nutrient restriction and crosstalk in metallostasis systems in microbial pathogens.Curr Opin Microbiol. 2020 Jun;55:17-25. doi: 10.1016/j.mib.2020.01.010. Epub 2020 Feb 12. Curr Opin Microbiol. 2020. PMID: 32062305 Free PMC article. Review.
-
Molecular Evolution of Transition Metal Bioavailability at the Host-Pathogen Interface.Trends Microbiol. 2021 May;29(5):441-457. doi: 10.1016/j.tim.2020.08.001. Epub 2020 Sep 18. Trends Microbiol. 2021. PMID: 32951986 Free PMC article. Review.
-
Metal homeostasis in infectious disease: recent advances in bacterial metallophores and the human metal-withholding response.Curr Opin Chem Biol. 2017 Apr;37:10-18. doi: 10.1016/j.cbpa.2016.09.012. Epub 2016 Dec 18. Curr Opin Chem Biol. 2017. PMID: 27992799 Free PMC article. Review.
Cited by
-
Zinc uptake system ZnuACB is essential for maintaining pathogenic phenotype of F4ac+ enterotoxigenic E. coli (ETEC) under a zinc restricted environment.Vet Res. 2020 Oct 7;51(1):127. doi: 10.1186/s13567-020-00854-1. Vet Res. 2020. PMID: 33028391 Free PMC article.
-
The C-Terminal Domain of Staphylococcus aureus Zinc Transport Protein AdcA Binds Plasminogen and Factor H In Vitro.Pathogens. 2022 Feb 12;11(2):240. doi: 10.3390/pathogens11020240. Pathogens. 2022. PMID: 35215183 Free PMC article.
-
Entropy redistribution controls allostery in a metalloregulatory protein.Proc Natl Acad Sci U S A. 2017 Apr 25;114(17):4424-4429. doi: 10.1073/pnas.1620665114. Epub 2017 Mar 27. Proc Natl Acad Sci U S A. 2017. PMID: 28348247 Free PMC article.
-
Zur: Zinc-Sensing Transcriptional Regulator in a Diverse Set of Bacterial Species.Pathogens. 2021 Mar 15;10(3):344. doi: 10.3390/pathogens10030344. Pathogens. 2021. PMID: 33804265 Free PMC article. Review.
-
Zur and zinc increase expression of E. coli ribosomal protein L31 through RNA-mediated repression of the repressor L31p.Nucleic Acids Res. 2022 Dec 9;50(22):12739-12753. doi: 10.1093/nar/gkac1086. Nucleic Acids Res. 2022. PMID: 36533433 Free PMC article.
References
-
- Andreini C., Banci L., Bertini I., and Rosato A. (2006) Counting the zinc-proteins encoded in the human genome. J. Proteome Res. 5, 196–201 - PubMed
-
- Outten C. E., and O'Halloran T. V. (2001) Femtomolar sensitivity of metalloregulatory proteins controlling zinc homeostasis. Science 292, 2488–2492 - PubMed
-
- Begg S. L., Eijkelkamp B. A., Luo Z., Couñago R. M., Morey J. R., Maher M. J., Ong C. L., McEwan A. G., Kobe B., O'Mara M. L., Paton J. C., and McDevitt C. A. (2015) Dysregulation of transition metal ion homeostasis is the molecular basis for cadmium toxicity in Streptococcus pneumoniae. Nat. Commun. 6, 6418. - PMC - PubMed
-
- Weinberg E. D. (1975) Nutritional immunity. Host's attempt to withold iron from microbial invaders. JAMA 231, 39–41 - PubMed
Publication types
MeSH terms
Substances
Associated data
- Actions
- Actions
- Actions
- Actions
- Actions
- Actions
- Actions
- Actions
- Actions
- Actions
- Actions
- Actions
- Actions
- Actions
- Actions
- Actions
Grants and funding
LinkOut - more resources
Full Text Sources
Other Literature Sources
Miscellaneous