A comprehensive transcriptional map of primate brain development
- PMID: 27409810
- PMCID: PMC5325728
- DOI: 10.1038/nature18637
A comprehensive transcriptional map of primate brain development
Abstract
The transcriptional underpinnings of brain development remain poorly understood, particularly in humans and closely related non-human primates. We describe a high-resolution transcriptional atlas of rhesus monkey (Macaca mulatta) brain development that combines dense temporal sampling of prenatal and postnatal periods with fine anatomical division of cortical and subcortical regions associated with human neuropsychiatric disease. Gene expression changes more rapidly before birth, both in progenitor cells and maturing neurons. Cortical layers and areas acquire adult-like molecular profiles surprisingly late in postnatal development. Disparate cell populations exhibit distinct developmental timing of gene expression, but also unexpected synchrony of processes underlying neural circuit construction including cell projection and adhesion. Candidate risk genes for neurodevelopmental disorders including primary microcephaly, autism spectrum disorder, intellectual disability, and schizophrenia show disease-specific spatiotemporal enrichment within developing neocortex. Human developmental expression trajectories are more similar to monkey than rodent, although approximately 9% of genes show human-specific regulation with evidence for prolonged maturation or neoteny compared to monkey.
Figures
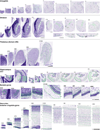
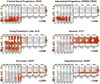
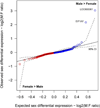
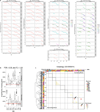
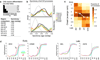
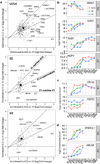
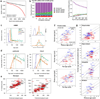
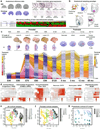
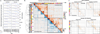
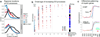
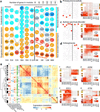
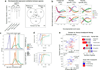
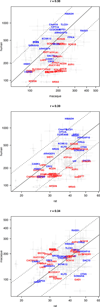
Similar articles
-
Spatiotemporal dynamics of the postnatal developing primate brain transcriptome.Hum Mol Genet. 2015 Aug 1;24(15):4327-39. doi: 10.1093/hmg/ddv166. Epub 2015 May 7. Hum Mol Genet. 2015. PMID: 25954031 Free PMC article.
-
Spatiotemporal transcriptomic divergence across human and macaque brain development.Science. 2018 Dec 14;362(6420):eaat8077. doi: 10.1126/science.aat8077. Epub 2018 Dec 13. Science. 2018. PMID: 30545855 Free PMC article.
-
Transcriptomic signatures of neuronal differentiation and their association with risk genes for autism spectrum and related neuropsychiatric disorders.Transl Psychiatry. 2016 Aug 2;6(8):e864. doi: 10.1038/tp.2016.119. Transl Psychiatry. 2016. PMID: 27483382 Free PMC article.
-
The Pleiotropic MET Receptor Network: Circuit Development and the Neural-Medical Interface of Autism.Biol Psychiatry. 2017 Mar 1;81(5):424-433. doi: 10.1016/j.biopsych.2016.08.035. Epub 2016 Sep 15. Biol Psychiatry. 2017. PMID: 27837921 Free PMC article. Review.
-
Transcriptional Regulators and Human-Specific/Primate-Specific Genes in Neocortical Neurogenesis.Int J Mol Sci. 2020 Jun 29;21(13):4614. doi: 10.3390/ijms21134614. Int J Mol Sci. 2020. PMID: 32610533 Free PMC article. Review.
Cited by
-
Evolution, Emotion, and Episodic Engagement.Am J Psychiatry. 2021 Aug 1;178(8):701-714. doi: 10.1176/appi.ajp.2020.20081187. Epub 2021 Jun 3. Am J Psychiatry. 2021. PMID: 34080889 Free PMC article. Review.
-
The road to precision psychiatry: translating genetics into disease mechanisms.Nat Neurosci. 2016 Oct 26;19(11):1397-1407. doi: 10.1038/nn.4409. Nat Neurosci. 2016. PMID: 27786179 Free PMC article. Review.
-
Transcription factor expression defines subclasses of developing projection neurons highly similar to single-cell RNA-seq subtypes.Proc Natl Acad Sci U S A. 2020 Oct 6;117(40):25074-25084. doi: 10.1073/pnas.2008013117. Epub 2020 Sep 18. Proc Natl Acad Sci U S A. 2020. PMID: 32948690 Free PMC article.
-
Opportunities and limitations of genetically modified nonhuman primate models for neuroscience research.Proc Natl Acad Sci U S A. 2020 Sep 29;117(39):24022-24031. doi: 10.1073/pnas.2006515117. Epub 2020 Aug 19. Proc Natl Acad Sci U S A. 2020. PMID: 32817435 Free PMC article. Review.
-
Laminar dynamics of deep projection neurons and mode of subplate formation are hallmarks of histogenetic subdivisions of the human cingulate cortex before onset of arealization.Brain Struct Funct. 2023 Mar;228(2):613-633. doi: 10.1007/s00429-022-02606-7. Epub 2023 Jan 2. Brain Struct Funct. 2023. PMID: 36592215 Free PMC article.
References
-
- Caldwell CA, Whiten Ain. Primates Perspect. Oxford University Press; 2011. pp. 652–662.
Publication types
MeSH terms
Grants and funding
LinkOut - more resources
Full Text Sources
Other Literature Sources