LD Motif Recognition by Talin: Structure of the Talin-DLC1 Complex
- PMID: 27265849
- PMCID: PMC4938799
- DOI: 10.1016/j.str.2016.04.016
LD Motif Recognition by Talin: Structure of the Talin-DLC1 Complex
Abstract
Cell migration requires coordination between integrin-mediated cell adhesion to the extracellular matrix and force applied to adhesion sites. Talin plays a key role in coupling integrin receptors to the actomyosin contractile machinery, while deleted in liver cancer 1 (DLC1) is a Rho GAP that binds talin and regulates Rho, and therefore actomyosin contractility. We show that the LD motif of DLC1 forms a helix that binds to the four-helix bundle of the talin R8 domain in a canonical triple-helix arrangement. We demonstrate that the same R8 surface interacts with the paxillin LD1 and LD2 motifs. We identify key charged residues that stabilize the R8 interactions with LD motifs and demonstrate their importance in vitro and in cells. Our results suggest a network of competitive interactions in adhesion complexes that involve LD motifs, and identify mutations that can be used to analyze the biological roles of specific protein-protein interactions in cell migration.
Copyright © 2016 The Author(s). Published by Elsevier Ltd.. All rights reserved.
Figures
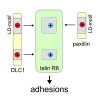
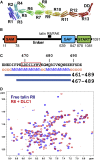
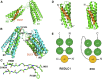
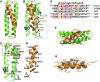
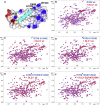
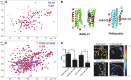
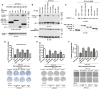
Similar articles
-
Full activity of the deleted in liver cancer 1 (DLC1) tumor suppressor depends on an LD-like motif that binds talin and focal adhesion kinase (FAK).Proc Natl Acad Sci U S A. 2011 Oct 11;108(41):17129-34. doi: 10.1073/pnas.1112122108. Epub 2011 Oct 3. Proc Natl Acad Sci U S A. 2011. PMID: 21969587 Free PMC article.
-
Mechanotransduction in talin through the interaction of the R8 domain with DLC1.PLoS Biol. 2018 Jul 20;16(7):e2005599. doi: 10.1371/journal.pbio.2005599. eCollection 2018 Jul. PLoS Biol. 2018. PMID: 30028837 Free PMC article.
-
CDK5 is a major regulator of the tumor suppressor DLC1.J Cell Biol. 2014 Dec 8;207(5):627-42. doi: 10.1083/jcb.201405105. Epub 2014 Dec 1. J Cell Biol. 2014. PMID: 25452387 Free PMC article.
-
Regulation of deleted in liver cancer 1 tumor suppressor by protein-protein interactions and phosphorylation.Int J Cancer. 2014 Jul 15;135(2):264-9. doi: 10.1002/ijc.28505. Epub 2013 Oct 17. Int J Cancer. 2014. PMID: 24114040 Review.
-
Integrin connections to the cytoskeleton through talin and vinculin.Biochem Soc Trans. 2008 Apr;36(Pt 2):235-9. doi: 10.1042/BST0360235. Biochem Soc Trans. 2008. PMID: 18363566 Review.
Cited by
-
Talin-KANK1 interaction controls the recruitment of cortical microtubule stabilizing complexes to focal adhesions.Elife. 2016 Jul 13;5:e18124. doi: 10.7554/eLife.18124. Elife. 2016. PMID: 27410476 Free PMC article.
-
Paxillin: A Hub for Mechano-Transduction from the β3 Integrin-Talin-Kindlin Axis.Front Cell Dev Biol. 2022 Apr 5;10:852016. doi: 10.3389/fcell.2022.852016. eCollection 2022. Front Cell Dev Biol. 2022. PMID: 35450290 Free PMC article. Review.
-
The role of single protein elasticity in mechanobiology.Nat Rev Mater. 2023 Jan;8:10-24. doi: 10.1038/s41578-022-00488-z. Epub 2022 Oct 24. Nat Rev Mater. 2023. PMID: 37469679 Free PMC article.
-
Paxillin phase separation promotes focal adhesion assembly and integrin signaling.J Cell Biol. 2024 Apr 1;223(4):e202209027. doi: 10.1083/jcb.202209027. Epub 2024 Mar 11. J Cell Biol. 2024. PMID: 38466167 Free PMC article.
-
Down-regulation of DLC1 in endothelial cells compromises the angiogenesis process.Cancer Lett. 2017 Jul 10;398:46-51. doi: 10.1016/j.canlet.2017.04.004. Epub 2017 Apr 10. Cancer Lett. 2017. PMID: 28408355 Free PMC article.
References
-
- Alam T., Alazmi M., Gao X., Arold S.T. How to find a leucine in a haystack? Structure, ligand recognition and regulation of leucine-aspartic acid (LD) motifs. Biochem. J. 2014;460:317–329. - PubMed
Publication types
MeSH terms
Substances
Grants and funding
LinkOut - more resources
Full Text Sources
Other Literature Sources
Research Materials
Miscellaneous