C9ORF72 poly(GA) aggregates sequester and impair HR23 and nucleocytoplasmic transport proteins
- PMID: 26998601
- PMCID: PMC5138863
- DOI: 10.1038/nn.4272
C9ORF72 poly(GA) aggregates sequester and impair HR23 and nucleocytoplasmic transport proteins
Abstract
Neuronal inclusions of poly(GA), a protein unconventionally translated from G4C2 repeat expansions in C9ORF72, are abundant in patients with frontotemporal dementia (FTD) and amyotrophic lateral sclerosis (ALS) caused by this mutation. To investigate poly(GA) toxicity, we generated mice that exhibit poly(GA) pathology, neurodegeneration and behavioral abnormalities reminiscent of FTD and ALS. These phenotypes occurred in the absence of TDP-43 pathology and required poly(GA) aggregation. HR23 proteins involved in proteasomal degradation and proteins involved in nucleocytoplasmic transport were sequestered by poly(GA) in these mice. HR23A and HR23B similarly colocalized to poly(GA) inclusions in C9ORF72 expansion carriers. Sequestration was accompanied by an accumulation of ubiquitinated proteins and decreased xeroderma pigmentosum C (XPC) levels in mice, indicative of HR23A and HR23B dysfunction. Restoring HR23B levels attenuated poly(GA) aggregation and rescued poly(GA)-induced toxicity in neuronal cultures. These data demonstrate that sequestration and impairment of nuclear HR23 and nucleocytoplasmic transport proteins is an outcome of, and a contributor to, poly(GA) pathology.
Conflict of interest statement
The authors declare no competing financial interests.
Figures
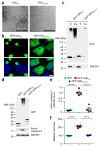
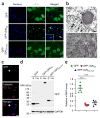
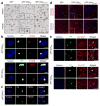
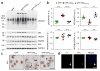
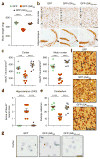
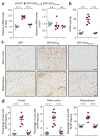
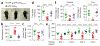
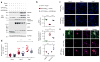
Similar articles
-
HR23B pathology preferentially co-localizes with p62, pTDP-43 and poly-GA in C9ORF72-linked frontotemporal dementia and amyotrophic lateral sclerosis.Acta Neuropathol Commun. 2019 Mar 13;7(1):39. doi: 10.1186/s40478-019-0694-6. Acta Neuropathol Commun. 2019. PMID: 30867060 Free PMC article.
-
Cytoplasmic poly-GA aggregates impair nuclear import of TDP-43 in C9orf72 ALS/FTLD.Hum Mol Genet. 2017 Feb 15;26(4):790-800. doi: 10.1093/hmg/ddw432. Hum Mol Genet. 2017. PMID: 28040728 Free PMC article.
-
Aberrant deposition of stress granule-resident proteins linked to C9orf72-associated TDP-43 proteinopathy.Mol Neurodegener. 2019 Feb 15;14(1):9. doi: 10.1186/s13024-019-0310-z. Mol Neurodegener. 2019. PMID: 30767771 Free PMC article.
-
An amyloid-like cascade hypothesis for C9orf72 ALS/FTD.Curr Opin Neurobiol. 2016 Feb;36:99-106. doi: 10.1016/j.conb.2015.10.009. Epub 2015 Nov 8. Curr Opin Neurobiol. 2016. PMID: 26555807 Review.
-
C9orf72 ALS-FTD: recent evidence for dysregulation of the autophagy-lysosome pathway at multiple levels.Autophagy. 2021 Nov;17(11):3306-3322. doi: 10.1080/15548627.2021.1872189. Epub 2021 Feb 26. Autophagy. 2021. PMID: 33632058 Free PMC article. Review.
Cited by
-
Congenic expression of poly-GA but not poly-PR in mice triggers selective neuron loss and interferon responses found in C9orf72 ALS.Acta Neuropathol. 2020 Aug;140(2):121-142. doi: 10.1007/s00401-020-02176-0. Epub 2020 Jun 19. Acta Neuropathol. 2020. PMID: 32562018 Free PMC article.
-
Neuronal UBE3A substrates hold therapeutic potential for Angelman syndrome.Curr Opin Neurobiol. 2024 Oct;88:102899. doi: 10.1016/j.conb.2024.102899. Epub 2024 Aug 9. Curr Opin Neurobiol. 2024. PMID: 39126903 Review.
-
PolyGA targets the ER stress-adaptive response by impairing GRP75 function at the MAM in C9ORF72-ALS/FTD.Acta Neuropathol. 2022 Nov;144(5):939-966. doi: 10.1007/s00401-022-02494-5. Epub 2022 Sep 19. Acta Neuropathol. 2022. PMID: 36121477 Free PMC article.
-
C9orf72-associated SMCR8 protein binds in the ubiquitin pathway and with proteins linked with neurological disease.Acta Neuropathol Commun. 2020 Jul 16;8(1):110. doi: 10.1186/s40478-020-00982-x. Acta Neuropathol Commun. 2020. PMID: 32678027 Free PMC article.
-
ALS' Perfect Storm: C9orf72-Associated Toxic Dipeptide Repeats as Potential Multipotent Disruptors of Protein Homeostasis.Cells. 2024 Jan 17;13(2):178. doi: 10.3390/cells13020178. Cells. 2024. PMID: 38247869 Free PMC article. Review.
References
MeSH terms
Substances
Grants and funding
- R01 NS094239/NS/NINDS NIH HHS/United States
- R21 NS089979/NS/NINDS NIH HHS/United States
- R01 NS093865/NS/NINDS NIH HHS/United States
- R21 NS079807/NS/NINDS NIH HHS/United States
- R01 NS087227/NS/NINDS NIH HHS/United States
- R01 NS088689/NS/NINDS NIH HHS/United States
- P01 NS084974/NS/NINDS NIH HHS/United States
- R21 NS084528/NS/NINDS NIH HHS/United States
- R01 ES020395/ES/NIEHS NIH HHS/United States
- U54 NS091046/NS/NINDS NIH HHS/United States
- R01 NS080882/NS/NINDS NIH HHS/United States
- P50 AG005131/AG/NIA NIH HHS/United States
- R01 NS085207/NS/NINDS NIH HHS/United States
- R01 NS063964/NS/NINDS NIH HHS/United States
- F32 NS087842/NS/NINDS NIH HHS/United States
- R01 AG026251/AG/NIA NIH HHS/United States
- R01 NS077402/NS/NINDS NIH HHS/United States
LinkOut - more resources
Full Text Sources
Other Literature Sources
Molecular Biology Databases
Miscellaneous