Building and re-building the heart by cardiomyocyte proliferation
- PMID: 26932668
- PMCID: PMC4813344
- DOI: 10.1242/dev.132910
Building and re-building the heart by cardiomyocyte proliferation
Abstract
The adult human heart does not regenerate significant amounts of lost tissue after injury. Rather than making new, functional muscle, human hearts are prone to scarring and hypertrophy, which can often lead to fatal arrhythmias and heart failure. The most-cited basis of this ineffective cardiac regeneration in mammals is the low proliferative capacity of adult cardiomyocytes. However, mammalian cardiomyocytes can avidly proliferate during fetal and neonatal development, and both adult zebrafish and neonatal mice can regenerate cardiac muscle after injury, suggesting that latent regenerative potential exists. Dissecting the cellular and molecular mechanisms that promote cardiomyocyte proliferation throughout life, deciphering why proliferative capacity normally dissipates in adult mammals, and deriving means to boost this capacity are primary goals in cardiovascular research. Here, we review our current understanding of how cardiomyocyte proliferation is regulated during heart development and regeneration.
Keywords: Cardiomyocyte; Heart regeneration; Proliferation.
© 2016. Published by The Company of Biologists Ltd.
Conflict of interest statement
The authors declare no competing or financial interests.
Figures
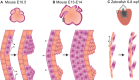
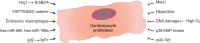
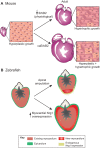
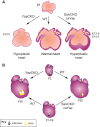
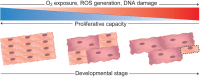
Similar articles
-
Mechanisms of Cardiac Regeneration.Dev Cell. 2016 Feb 22;36(4):362-74. doi: 10.1016/j.devcel.2016.01.018. Dev Cell. 2016. PMID: 26906733 Free PMC article. Review.
-
Cardiomyocyte proliferation in cardiac development and regeneration: a guide to methodologies and interpretations.Am J Physiol Heart Circ Physiol. 2015 Oct;309(8):H1237-50. doi: 10.1152/ajpheart.00559.2015. Epub 2015 Sep 4. Am J Physiol Heart Circ Physiol. 2015. PMID: 26342071 Review.
-
Cardiomyocyte proliferation in zebrafish and mammals: lessons for human disease.Cell Mol Life Sci. 2017 Apr;74(8):1367-1378. doi: 10.1007/s00018-016-2404-x. Epub 2016 Nov 3. Cell Mol Life Sci. 2017. PMID: 27812722 Free PMC article. Review.
-
Polyploidy in Cardiomyocytes: Roadblock to Heart Regeneration?Circ Res. 2020 Feb 14;126(4):552-565. doi: 10.1161/CIRCRESAHA.119.315408. Epub 2020 Feb 13. Circ Res. 2020. PMID: 32078450 Review.
-
Cellular and Molecular Mechanism of Cardiac Regeneration: A Comparison of Newts, Zebrafish, and Mammals.Biomolecules. 2020 Aug 19;10(9):1204. doi: 10.3390/biom10091204. Biomolecules. 2020. PMID: 32825069 Free PMC article. Review.
Cited by
-
Immunity in salamander regeneration: Where are we standing and where are we headed?Dev Dyn. 2021 Jun;250(6):753-767. doi: 10.1002/dvdy.251. Epub 2020 Sep 21. Dev Dyn. 2021. PMID: 32924213 Free PMC article. Review.
-
MiR-21, MiR-29a, GATA4, and MEF2c Expression Changes in Endothelin-1 and Angiotensin II Cardiac Hypertrophy Stimulated Isl-1+Sca-1+c-kit+ Porcine Cardiac Progenitor Cells In Vitro.Cells. 2019 Nov 9;8(11):1416. doi: 10.3390/cells8111416. Cells. 2019. PMID: 31717562 Free PMC article.
-
Metabolic Pathway of Cardiospecific Troponins: From Fundamental Aspects to Diagnostic Role (Comprehensive Review).Front Mol Biosci. 2022 Apr 19;9:841277. doi: 10.3389/fmolb.2022.841277. eCollection 2022. Front Mol Biosci. 2022. PMID: 35517866 Free PMC article. Review.
-
Adult Cardiomyocyte Cell Cycle Detour: Off-ramp to Quiescent Destinations.Trends Endocrinol Metab. 2019 Aug;30(8):557-567. doi: 10.1016/j.tem.2019.05.006. Epub 2019 Jun 28. Trends Endocrinol Metab. 2019. PMID: 31262545 Free PMC article. Review.
-
Stimulation of Cardiomyocyte Proliferation Is Dependent on Species and Level of Maturation.Front Cell Dev Biol. 2022 May 19;10:806564. doi: 10.3389/fcell.2022.806564. eCollection 2022. Front Cell Dev Biol. 2022. PMID: 35663393 Free PMC article.
References
-
- Bär C., de Jesus B. B., Serrano R., Tejera A., Ayuso E., Jimenez V., Formentini I., Bobadilla M., Mizrahi J., de Martino A. et al. (2014). Telomerase expression confers cardioprotection in the adult mouse heart after acute myocardial infarction. Nat. Commun. 5, 5863 10.1038/ncomms6863 - DOI - PMC - PubMed
Publication types
MeSH terms
Substances
Grants and funding
LinkOut - more resources
Full Text Sources
Other Literature Sources