Kissing-loop interaction between 5' and 3' ends of tick-borne Langat virus genome 'bridges the gap' between mosquito- and tick-borne flaviviruses in mechanisms of viral RNA cyclization: applications for virus attenuation and vaccine development
- PMID: 26850640
- PMCID: PMC4838367
- DOI: 10.1093/nar/gkw061
Kissing-loop interaction between 5' and 3' ends of tick-borne Langat virus genome 'bridges the gap' between mosquito- and tick-borne flaviviruses in mechanisms of viral RNA cyclization: applications for virus attenuation and vaccine development
Abstract
Insertion of microRNA target sequences into the flavivirus genome results in selective tissue-specific attenuation and host-range restriction of live attenuated vaccine viruses. However, previous strategies for miRNA-targeting did not incorporate a mechanism to prevent target elimination under miRNA-mediated selective pressure, restricting their use in vaccine development. To overcome this limitation, we developed a new approach for miRNA-targeting of tick-borne flavivirus (Langat virus, LGTV) in the duplicated capsid gene region (DCGR). Genetic stability of viruses with DCGR was ensured by the presence of multiple cis-acting elements within the N-terminal capsid coding region, including the stem-loop structure (5'SL6) at the 3' end of the promoter. We found that the 5'SL6 functions as a structural scaffold for the conserved hexanucleotide motif at its tip and engages in a complementary interaction with the region present in the 3' NCR to enhance viral RNA replication. The resulting kissing-loop interaction, common in tick-borne flaviviruses, supports a single pair of cyclization elements (CYC) and functions as a homolog of the second pair of CYC that is present in the majority of mosquito-borne flaviviruses. Placing miRNA targets into the DCGR results in superior attenuation of LGTV in the CNS and does not interfere with development of protective immunity in immunized mice.
Published by Oxford University Press on behalf of Nucleic Acids Research 2016. This work is written by (a) US Government employee(s) and is in the public domain in the US.
Figures
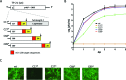
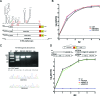
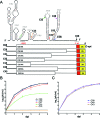
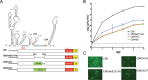
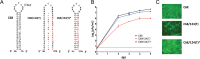
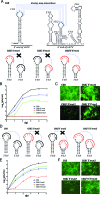
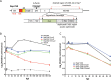
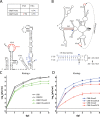
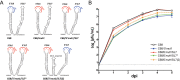
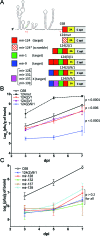
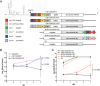
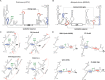
Similar articles
-
Synergistic Internal Ribosome Entry Site/MicroRNA-Based Approach for Flavivirus Attenuation and Live Vaccine Development.mBio. 2017 Apr 18;8(2):e02326-16. doi: 10.1128/mBio.02326-16. mBio. 2017. PMID: 28420742 Free PMC article.
-
Tick-borne Langat/mosquito-borne dengue flavivirus chimera, a candidate live attenuated vaccine for protection against disease caused by members of the tick-borne encephalitis virus complex: evaluation in rhesus monkeys and in mosquitoes.J Virol. 2001 Sep;75(17):8259-67. doi: 10.1128/jvi.75.17.8259-8267.2001. J Virol. 2001. PMID: 11483771 Free PMC article.
-
MicroRNA-based control of tick-borne flavivirus neuropathogenesis: Challenges and perspectives.Antiviral Res. 2016 Mar;127:57-67. doi: 10.1016/j.antiviral.2016.01.003. Epub 2016 Jan 19. Antiviral Res. 2016. PMID: 26794396 Free PMC article.
-
Steps of the tick-borne encephalitis virus replication cycle that affect neuropathogenesis.Virus Res. 2005 Aug;111(2):161-74. doi: 10.1016/j.virusres.2005.04.007. Virus Res. 2005. PMID: 15871909 Review.
-
Flavivirus immunization with capsid-deletion mutants: basics, benefits, and barriers.Viral Immunol. 2004;17(4):461-72. doi: 10.1089/vim.2004.17.461. Viral Immunol. 2004. PMID: 15671744 Review.
Cited by
-
Stable and Highly Immunogenic MicroRNA-Targeted Single-Dose Live Attenuated Vaccine Candidate against Tick-Borne Encephalitis Constructed Using Genetic Backbone of Langat Virus.mBio. 2019 Apr 23;10(2):e02904-18. doi: 10.1128/mBio.02904-18. mBio. 2019. PMID: 31015334 Free PMC article.
-
Information Encoded by the Flavivirus Genomes beyond the Nucleotide Sequence.Int J Mol Sci. 2021 Apr 3;22(7):3738. doi: 10.3390/ijms22073738. Int J Mol Sci. 2021. PMID: 33916729 Free PMC article. Review.
-
Routes of Zika virus dissemination in the testis and epididymis of immunodeficient mice.Nat Commun. 2018 Dec 17;9(1):5350. doi: 10.1038/s41467-018-07782-x. Nat Commun. 2018. PMID: 30559387 Free PMC article.
-
Breast milk transmission and involvement of mammary glands in tick-borne flavivirus infected mice.J Virol. 2024 Mar 19;98(3):e0170923. doi: 10.1128/jvi.01709-23. Epub 2024 Feb 2. J Virol. 2024. PMID: 38305156 Free PMC article.
-
Novel Approach for Insertion of Heterologous Sequences into Full-Length ZIKV Genome Results in Superior Level of Gene Expression and Insert Stability.Viruses. 2020 Jan 3;12(1):61. doi: 10.3390/v12010061. Viruses. 2020. PMID: 31947825 Free PMC article.
References
-
- Lasala P.R., Holbrook M. Tick-borne flaviviruses. Clin. Lab. Med. 2010;30:221–235. - PubMed
-
- Gritsun T.S., Lashkevich V.A., Gould E.A. Tick-borne encephalitis. Antiviral Res. 2003;57:129–146. - PubMed
-
- Smith C.E. A virus resembling Russian spring-summer encephalitis virus from an ixodid tick in Malaya. Nature. 1956;178:581–582. - PubMed
Publication types
MeSH terms
Substances
Grants and funding
LinkOut - more resources
Full Text Sources
Other Literature Sources
Miscellaneous