Synonymous Codons Direct Cotranslational Folding toward Different Protein Conformations
- PMID: 26849192
- PMCID: PMC4745992
- DOI: 10.1016/j.molcel.2016.01.008
Synonymous Codons Direct Cotranslational Folding toward Different Protein Conformations
Abstract
In all genomes, most amino acids are encoded by more than one codon. Synonymous codons can modulate protein production and folding, but the mechanism connecting codon usage to protein homeostasis is not known. Here we show that synonymous codon variants in the gene encoding gamma-B crystallin, a mammalian eye-lens protein, modulate the rates of translation and cotranslational folding of protein domains monitored in real time by Förster resonance energy transfer and fluorescence-intensity changes. Gamma-B crystallins produced from mRNAs with changed codon bias have the same amino acid sequence but attain different conformations, as indicated by altered in vivo stability and in vitro protease resistance. 2D NMR spectroscopic data suggest that structural differences are associated with different cysteine oxidation states of the purified proteins, providing a link between translation, folding, and the structures of isolated proteins. Thus, synonymous codons provide a secondary code for protein folding in the cell.
Copyright © 2016 Elsevier Inc. All rights reserved.
Figures
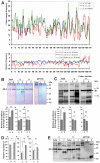
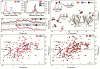
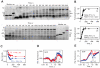
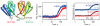
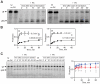
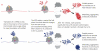
Similar articles
-
A code within the genetic code: codon usage regulates co-translational protein folding.Cell Commun Signal. 2020 Sep 9;18(1):145. doi: 10.1186/s12964-020-00642-6. Cell Commun Signal. 2020. PMID: 32907610 Free PMC article. Review.
-
Synonymous but Not Silent: The Codon Usage Code for Gene Expression and Protein Folding.Annu Rev Biochem. 2021 Jun 20;90:375-401. doi: 10.1146/annurev-biochem-071320-112701. Epub 2021 Jan 13. Annu Rev Biochem. 2021. PMID: 33441035 Free PMC article.
-
Synonymous codon substitutions perturb cotranslational protein folding in vivo and impair cell fitness.Proc Natl Acad Sci U S A. 2020 Feb 18;117(7):3528-3534. doi: 10.1073/pnas.1907126117. Epub 2020 Feb 3. Proc Natl Acad Sci U S A. 2020. PMID: 32015130 Free PMC article.
-
Translation Rates and Protein Folding.J Mol Biol. 2024 Jul 15;436(14):168384. doi: 10.1016/j.jmb.2023.168384. Epub 2023 Dec 6. J Mol Biol. 2024. PMID: 38065274 Review.
-
A Code Within a Code: How Codons Fine-Tune Protein Folding in the Cell.Biochemistry (Mosc). 2021 Aug;86(8):976-991. doi: 10.1134/S0006297921080083. Biochemistry (Mosc). 2021. PMID: 34488574 Free PMC article. Review.
Cited by
-
Synonymous codon usage regulates translation initiation.Cell Rep. 2023 Dec 26;42(12):113413. doi: 10.1016/j.celrep.2023.113413. Epub 2023 Dec 12. Cell Rep. 2023. PMID: 38096059 Free PMC article.
-
Analysis of 3.5 million SARS-CoV-2 sequences reveals unique mutational trends with consistent nucleotide and codon frequencies.Virol J. 2023 Feb 17;20(1):31. doi: 10.1186/s12985-023-01982-8. Virol J. 2023. PMID: 36812119 Free PMC article.
-
The codon sequences predict protein lifetimes and other parameters of the protein life cycle in the mouse brain.Sci Rep. 2018 Nov 15;8(1):16913. doi: 10.1038/s41598-018-35277-8. Sci Rep. 2018. PMID: 30443017 Free PMC article.
-
Conservation of location of several specific inhibitory codon pairs in the Saccharomyces sensu stricto yeasts reveals translational selection.Nucleic Acids Res. 2019 Feb 20;47(3):1164-1177. doi: 10.1093/nar/gky1262. Nucleic Acids Res. 2019. PMID: 30576464 Free PMC article.
-
Analysis of codon usage patterns in open reading frame 4 of hepatitis E viruses.Beni Suef Univ J Basic Appl Sci. 2022;11(1):65. doi: 10.1186/s43088-022-00244-w. Epub 2022 May 10. Beni Suef Univ J Basic Appl Sci. 2022. PMID: 35573872 Free PMC article.
References
-
- Bloemendal H, de Jong W, Jaenicke R, Lubsen NH, Slingsby C, Tardieu A. Ageing and vision: structure, stability and function of lens crystallins. Prog. Biophys. Mol. Biol. 2004;86:407–485. - PubMed
-
- Buchan JR, Stansfield I. Halting a cellular production line: responses to ribosomal pausing during translation. Biol. Cell. 2007;99:475–487. - PubMed
-
- Cabrita LD, Dobson CM, Christodoulou J. Protein folding on the ribosome. Curr. Opin. Struct. Biol. 2010;20:33–45. - PubMed
Publication types
MeSH terms
Substances
Grants and funding
LinkOut - more resources
Full Text Sources
Other Literature Sources