Prions and Protein Assemblies that Convey Biological Information in Health and Disease
- PMID: 26844828
- PMCID: PMC4748384
- DOI: 10.1016/j.neuron.2016.01.026
Prions and Protein Assemblies that Convey Biological Information in Health and Disease
Abstract
Prions derived from the prion protein (PrP) were first characterized as infectious agents that transmit pathology between individuals. However, the majority of cases of neurodegeneration caused by PrP prions occur sporadically. Proteins that self-assemble as cross-beta sheet amyloids are a defining pathological feature of infectious prion disorders and all major age-associated neurodegenerative diseases. In fact, multiple non-infectious proteins exhibit properties of template-driven self-assembly that are strikingly similar to PrP. Evidence suggests that like PrP, many proteins form aggregates that propagate between cells and convert cognate monomer into ordered assemblies. We now recognize that numerous proteins assemble into macromolecular complexes as part of normal physiology, some of which are self-amplifying. This review highlights similarities among infectious and non-infectious neurodegenerative diseases associated with prions, emphasizing the normal and pathogenic roles of higher-order protein assemblies. We propose that studies of the structural and cellular biology of pathological versus physiological aggregates will be mutually informative.
Copyright © 2016 Elsevier Inc. All rights reserved.
Figures
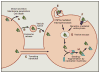
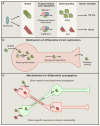
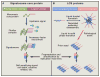
Similar articles
-
Synthetic prions and other human neurodegenerative proteinopathies.Virus Res. 2015 Sep 2;207:25-37. doi: 10.1016/j.virusres.2014.10.020. Epub 2014 Oct 31. Virus Res. 2015. PMID: 25449570 Review.
-
[Can prion-like propagation occur in neurodegenerative diseases?: in view of transmissible systemic amyloidosis].Brain Nerve. 2012 Jun;64(6):665-74. Brain Nerve. 2012. PMID: 22647474 Review. Japanese.
-
Prions and Non-infectious Amyloids of Mammals - Similarities and Differences.Biochemistry (Mosc). 2018 Oct;83(10):1184-1195. doi: 10.1134/S0006297918100048. Biochemistry (Mosc). 2018. PMID: 30472956 Review.
-
The cell biology of prion-like spread of protein aggregates: mechanisms and implication in neurodegeneration.Biochem J. 2013 May 15;452(1):1-17. doi: 10.1042/BJ20121898. Biochem J. 2013. PMID: 23614720 Review.
-
Neuroprotective and neurotoxic signaling by the prion protein.Top Curr Chem. 2011;305:101-19. doi: 10.1007/128_2011_160. Top Curr Chem. 2011. PMID: 21598098 Review.
Cited by
-
Populations of Tau Conformers Drive Prion-like Strain Effects in Alzheimer's Disease and Related Dementias.Cells. 2022 Sep 26;11(19):2997. doi: 10.3390/cells11192997. Cells. 2022. PMID: 36230957 Free PMC article. Review.
-
Detection of TAR DNA-binding protein 43 (TDP-43) oligomers as initial intermediate species during aggregate formation.J Biol Chem. 2019 Apr 26;294(17):6696-6709. doi: 10.1074/jbc.RA118.005889. Epub 2019 Mar 1. J Biol Chem. 2019. PMID: 30824544 Free PMC article.
-
Defects in trafficking bridge Parkinson's disease pathology and genetics.Nature. 2016 Nov 10;539(7628):207-216. doi: 10.1038/nature20414. Nature. 2016. PMID: 27830778 Review.
-
Amyloid-β accumulation in the CNS in human growth hormone recipients in the UK.Acta Neuropathol. 2017 Aug;134(2):221-240. doi: 10.1007/s00401-017-1703-0. Epub 2017 Mar 27. Acta Neuropathol. 2017. PMID: 28349199 Free PMC article.
-
Phosphorylation at Ser8 as an Intrinsic Regulatory Switch to Regulate the Morphologies and Structures of Alzheimer's 40-residue β-Amyloid (Aβ40) Fibrils.J Biol Chem. 2017 Feb 17;292(7):2611-2623. doi: 10.1074/jbc.M116.757179. Epub 2016 Dec 28. J Biol Chem. 2017. PMID: 28031462 Free PMC article.
References
-
- Aguzzi A, Rajendran L. The transcellular spread of cytosolic amyloids, prions, and prionoids. Neuron. 2009;64:783–790. - PubMed
-
- Ahmed Z, Cooper J, Murray TK, Garn K, McNaughton E, Clarke H, Parhizkar S, Ward MA, Cavallini A, Jackson S, et al. A novel in vivo model of tau propagation with rapid and progressive neurofibrillary tangle pathology: the pattern of spread is determined by connectivity, not proximity. Acta Neuropathol. 2014;127:667–683. - PMC - PubMed
-
- Alonso ADC, Li B, Grundke-Iqbal I, Iqbal K. Mechanism of tau-induced neurodegeneration in Alzheimer disease and related tauopathies. Curr Alzheimer Res. 2008;5:375–384. - PubMed
Publication types
MeSH terms
Substances
Grants and funding
LinkOut - more resources
Full Text Sources
Other Literature Sources
Medical
Molecular Biology Databases
Research Materials