Phosphoproteomics reveals that Parkinson's disease kinase LRRK2 regulates a subset of Rab GTPases
- PMID: 26824392
- PMCID: PMC4769169
- DOI: 10.7554/eLife.12813
Phosphoproteomics reveals that Parkinson's disease kinase LRRK2 regulates a subset of Rab GTPases
Abstract
Mutations in Park8, encoding for the multidomain Leucine-rich repeat kinase 2 (LRRK2) protein, comprise the predominant genetic cause of Parkinson's disease (PD). G2019S, the most common amino acid substitution activates the kinase two- to threefold. This has motivated the development of LRRK2 kinase inhibitors; however, poor consensus on physiological LRRK2 substrates has hampered clinical development of such therapeutics. We employ a combination of phosphoproteomics, genetics, and pharmacology to unambiguously identify a subset of Rab GTPases as key LRRK2 substrates. LRRK2 directly phosphorylates these both in vivo and in vitro on an evolutionary conserved residue in the switch II domain. Pathogenic LRRK2 variants mapping to different functional domains increase phosphorylation of Rabs and this strongly decreases their affinity to regulatory proteins including Rab GDP dissociation inhibitors (GDIs). Our findings uncover a key class of bona-fide LRRK2 substrates and a novel regulatory mechanism of Rabs that connects them to PD.
Keywords: LRRK2; biochemistry; cell biology; chemical proteomics; human; mouse; neurodegeneration; parkinson-s disease; phosphoproteomics; rabs.
Conflict of interest statement
The other authors declare that no competing interests exist.
SWi: Employee of GlaxoSmithKline, a global healthcare company that may conceivably benefit financially through this publication.
MJF: Employee of Merck Research Laboratories.
JAM: Employee of GlaxoSmithKline, a global healthcare company that may conceivably benefit financially through this publication.
ADR: Employee of Merck Research Laboratories, Contributed unpublished essential data or reagents.
Figures
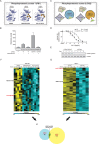
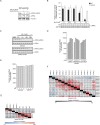
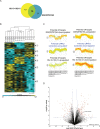
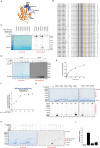
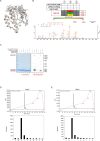
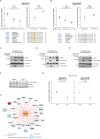
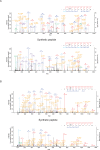
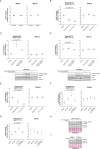
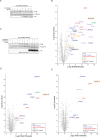
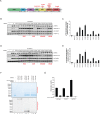
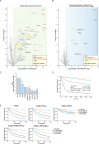
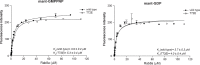
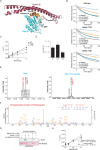
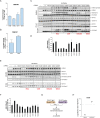
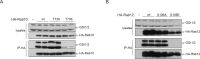
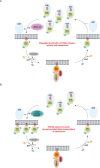
Comment in
-
Parkinson's Disease: A Traffic Jam?Curr Biol. 2016 Apr 25;26(8):R332-4. doi: 10.1016/j.cub.2016.03.001. Curr Biol. 2016. PMID: 27115692
-
Identification of bona-fide LRRK2 kinase substrates.Mov Disord. 2016 Aug;31(8):1140-1. doi: 10.1002/mds.26647. Epub 2016 Apr 29. Mov Disord. 2016. PMID: 27126091 Free PMC article. No abstract available.
Similar articles
-
Dysregulated phosphorylation of Rab GTPases by LRRK2 induces neurodegeneration.Mol Neurodegener. 2018 Feb 13;13(1):8. doi: 10.1186/s13024-018-0240-1. Mol Neurodegener. 2018. PMID: 29439717 Free PMC article.
-
LRRK2 and Rab GTPases.Biochem Soc Trans. 2018 Dec 17;46(6):1707-1712. doi: 10.1042/BST20180470. Epub 2018 Nov 22. Biochem Soc Trans. 2018. PMID: 30467121 Review.
-
LRRK2 phosphorylation of Rab GTPases in Parkinson's disease.FEBS Lett. 2023 Mar;597(6):811-818. doi: 10.1002/1873-3468.14492. Epub 2022 Sep 26. FEBS Lett. 2023. PMID: 36114007 Review.
-
Development of phospho-specific Rab protein antibodies to monitor in vivo activity of the LRRK2 Parkinson's disease kinase.Biochem J. 2018 Jan 2;475(1):1-22. doi: 10.1042/BCJ20170802. Biochem J. 2018. PMID: 29127256 Free PMC article.
-
Deciphering the LRRK code: LRRK1 and LRRK2 phosphorylate distinct Rab proteins and are regulated by diverse mechanisms.Biochem J. 2021 Feb 12;478(3):553-578. doi: 10.1042/BCJ20200937. Biochem J. 2021. PMID: 33459343 Free PMC article.
Cited by
-
Molecular mechanisms of polarized transport to the apical plasma membrane.Front Cell Dev Biol. 2024 Sep 26;12:1477173. doi: 10.3389/fcell.2024.1477173. eCollection 2024. Front Cell Dev Biol. 2024. PMID: 39445332 Free PMC article. Review.
-
System-wide identification and prioritization of enzyme substrates by thermal analysis.Nat Commun. 2021 Feb 26;12(1):1296. doi: 10.1038/s41467-021-21540-6. Nat Commun. 2021. PMID: 33637753 Free PMC article.
-
LRRK2 and the Endolysosomal System in Parkinson's Disease.J Parkinsons Dis. 2020;10(4):1271-1291. doi: 10.3233/JPD-202138. J Parkinsons Dis. 2020. PMID: 33044192 Free PMC article. Review.
-
Rac1-PAK1 regulation of Rab11 cycling promotes junction destabilization.J Cell Biol. 2021 Jun 7;220(6):e202002114. doi: 10.1083/jcb.202002114. J Cell Biol. 2021. PMID: 33914026 Free PMC article.
-
The In Situ Structure of Parkinson's Disease-Linked LRRK2.Cell. 2020 Sep 17;182(6):1508-1518.e16. doi: 10.1016/j.cell.2020.08.004. Epub 2020 Aug 11. Cell. 2020. PMID: 32783917 Free PMC article.
References
-
- Baptista MAS, Dave KD, Frasier MA, Sherer TB, Greeley M, Beck MJ, Varsho JS, Parker GA, Moore C, Churchill MJ, Meshul CK, Fiske BK. Loss of leucine-rich repeat kinase 2 (LRRK2) in rats leads to progressive abnormal phenotypes in peripheral organs. PLoS One. 2013;8:e12813. doi: 10.1371/journal.pone.0080705. - DOI - PMC - PubMed
Publication types
MeSH terms
Substances
Grants and funding
LinkOut - more resources
Full Text Sources
Other Literature Sources
Medical
Molecular Biology Databases
Research Materials