Pro-inflammatory Macrophages Sustain Pyruvate Oxidation through Pyruvate Dehydrogenase for the Synthesis of Itaconate and to Enable Cytokine Expression
- PMID: 26679997
- PMCID: PMC4759172
- DOI: 10.1074/jbc.M115.676817
Pro-inflammatory Macrophages Sustain Pyruvate Oxidation through Pyruvate Dehydrogenase for the Synthesis of Itaconate and to Enable Cytokine Expression
Abstract
Upon stimulation with Th1 cytokines or bacterial lipopolysaccharides, resting macrophages shift their phenotype toward a pro-inflammatory state as part of the innate immune response. LPS-activated macrophages undergo profound metabolic changes to adapt to these new physiological requirements. One key step to mediate this metabolic adaptation is the stabilization of HIF1α, which leads to increased glycolysis and lactate release, as well as decreased oxygen consumption. HIF1 abundance can result in the induction of the gene encoding pyruvate dehydrogenase kinase 1 (PDK1), which inhibits pyruvate dehydrogenase (PDH) via phosphorylation. Therefore, it has been speculated that pyruvate oxidation through PDH is decreased in pro-inflammatory macrophages. However, to answer this open question, an in-depth analysis of this metabolic branching point was so far lacking. In this work, we applied stable isotope-assisted metabolomics techniques and demonstrate that pyruvate oxidation is maintained in mature pro-inflammatory macrophages. Glucose-derived pyruvate is oxidized via PDH to generate citrate in the mitochondria. Citrate is used for the synthesis of the antimicrobial metabolite itaconate and for lipogenesis. An increased demand for these metabolites decreases citrate oxidation through the tricarboxylic acid cycle, whereas increased glutamine uptake serves to replenish the TCA cycle. Furthermore, we found that the PDH flux is maintained by unchanged PDK1 abundance, despite the presence of HIF1. By pharmacological intervention, we demonstrate that the PDH flux is an important node for M(LPS) macrophage activation. Therefore, PDH represents a metabolic intervention point that might become a research target for translational medicine to treat chronic inflammatory diseases.
Keywords: Itaconate; immunology; inflammation; macrophage; metabolic regulation; metabolism; mitochondrial metabolism; pyruvate;.
© 2016 by The American Society for Biochemistry and Molecular Biology, Inc.
Figures
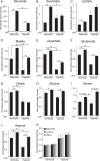
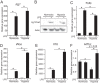
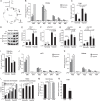
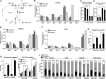
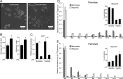
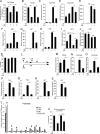
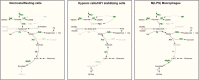
Similar articles
-
Metabolic Connection of Inflammatory Pain: Pivotal Role of a Pyruvate Dehydrogenase Kinase-Pyruvate Dehydrogenase-Lactic Acid Axis.J Neurosci. 2015 Oct 21;35(42):14353-69. doi: 10.1523/JNEUROSCI.1910-15.2015. J Neurosci. 2015. PMID: 26490872 Free PMC article.
-
Stimulating pyruvate dehydrogenase complex reduces itaconate levels and enhances TCA cycle anabolic bioenergetics in acutely inflamed monocytes.J Leukoc Biol. 2020 Mar;107(3):467-484. doi: 10.1002/JLB.3A1119-236R. Epub 2020 Jan 2. J Leukoc Biol. 2020. PMID: 31894617 Free PMC article.
-
Metabolic reorganization in winter: Regulation of pyruvate dehydrogenase (PDH) during long-term freezing and anoxia.Cryobiology. 2019 Feb;86:10-18. doi: 10.1016/j.cryobiol.2019.01.006. Epub 2019 Jan 10. Cryobiology. 2019. PMID: 30639451
-
Itaconate: an emerging determinant of inflammation in activated macrophages.Immunol Cell Biol. 2019 Feb;97(2):134-141. doi: 10.1111/imcb.12218. Epub 2018 Dec 11. Immunol Cell Biol. 2019. PMID: 30428148 Review.
-
Sepsis, pyruvate, and mitochondria energy supply chain shortage.J Leukoc Biol. 2022 Dec;112(6):1509-1514. doi: 10.1002/JLB.3MR0322-692RR. Epub 2022 Jul 22. J Leukoc Biol. 2022. PMID: 35866365 Free PMC article. Review.
Cited by
-
Decorin promotes decidual M1-like macrophage polarization via mitochondrial dysfunction resulting in recurrent pregnancy loss.Theranostics. 2022 Oct 17;12(17):7216-7236. doi: 10.7150/thno.78467. eCollection 2022. Theranostics. 2022. PMID: 36438479 Free PMC article.
-
Role of metabolic reprogramming in pro-inflammatory cytokine secretion from LPS or silica-activated macrophages.Front Immunol. 2022 Oct 21;13:936167. doi: 10.3389/fimmu.2022.936167. eCollection 2022. Front Immunol. 2022. PMID: 36341426 Free PMC article. Review.
-
Acute neuroinflammation promotes a metabolic shift that alters extracellular vesicle cargo in the mouse brain cortex.J Extracell Biol. 2024 Jun 27;3(7):e165. doi: 10.1002/jex2.165. eCollection 2024 Jul. J Extracell Biol. 2024. PMID: 38947878 Free PMC article.
-
The Metabolic Signature of Macrophage Responses.Front Immunol. 2019 Jul 3;10:1462. doi: 10.3389/fimmu.2019.01462. eCollection 2019. Front Immunol. 2019. PMID: 31333642 Free PMC article. Review.
-
Metabolism in atherosclerotic plaques: immunoregulatory mechanisms in the arterial wall.Clin Sci (Lond). 2022 Mar 31;136(6):435-454. doi: 10.1042/CS20201293. Clin Sci (Lond). 2022. PMID: 35348183 Free PMC article. Review.
References
-
- Gordon S., and Taylor P. R. (2005) Monocyte and macrophage heterogeneity. Nat. Rev. Immunol. 5, 953–964 - PubMed
-
- Murray P. J., Allen J. E., Biswas S. K., Fisher E. A., Gilroy D. W., Goerdt S., Gordon S., Hamilton J. A., Ivashkiv L. B., Lawrence T., Locati M., Mantovani A., Martinez F. O., Mege J. L., Mosser D. M., et al. (2014) Macrophage activation and polarization: nomenclature and experimental guidelines. Immunity 41, 14. - PMC - PubMed
-
- Basler T., Jeckstadt S., Valentin-Weigand P., and Goethe R. (2006) Mycobacterium paratuberculosis, Mycobacterium smegmatis, and lipopolysaccharide induce different transcriptional and post-transcriptional regulation of the IRG1 gene in murine macrophages. J. Leukocyte Biol. 79, 628–638 - PubMed
-
- Degrandi D., Hoffmann R., Beuter-Gunia C., and Pfeffer K. (2009) The proinflammatory cytokine-induced IRG1 protein associates with mitochondria. J. Interferon Cytokine Res. 29, 55–67 - PubMed
Publication types
MeSH terms
Substances
LinkOut - more resources
Full Text Sources
Molecular Biology Databases
Miscellaneous