Global Analysis of mRNA, Translation, and Protein Localization: Local Translation Is a Key Regulator of Cell Protrusions
- PMID: 26555054
- PMCID: PMC4643311
- DOI: 10.1016/j.devcel.2015.10.005
Global Analysis of mRNA, Translation, and Protein Localization: Local Translation Is a Key Regulator of Cell Protrusions
Abstract
Polarization of cells into a protrusive front and a retracting cell body is the hallmark of mesenchymal-like cell migration. Many mRNAs are localized to protrusions, but it is unclear to what degree mRNA localization contributes toward protrusion formation. We performed global quantitative analysis of the distributions of mRNAs, proteins, and translation rates between protrusions and the cell body by RNA sequencing (RNA-seq) and quantitative proteomics. Our results reveal local translation as a key determinant of protein localization to protrusions. Accordingly, inhibition of local translation destabilizes protrusions and inhibits mesenchymal-like morphology. Interestingly, many mRNAs localized to protrusions are translationally repressed. Specific cis-regulatory elements within mRNA UTRs define whether mRNAs are locally translated or repressed. Finally, RNAi screening of RNA-binding proteins (RBPs) enriched in protrusions revealed trans-regulators of localized translation that are functionally important for protrusions. We propose that by deciphering the localized mRNA UTR code, these proteins regulate protrusion stability and mesenchymal-like morphology.
Copyright © 2015 The Authors. Published by Elsevier Inc. All rights reserved.
Figures
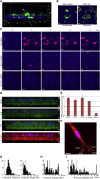
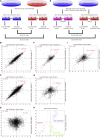
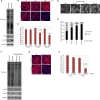
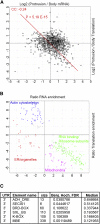
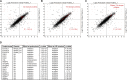
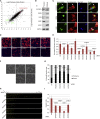
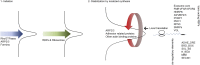
Similar articles
-
Translational regulation of protrusion-localized RNAs involves silencing and clustering after transport.Elife. 2019 Jul 10;8:e44752. doi: 10.7554/eLife.44752. Elife. 2019. PMID: 31290739 Free PMC article.
-
Subcellular mRNA Localization Regulates Ribosome Biogenesis in Migrating Cells.Dev Cell. 2020 Nov 9;55(3):298-313.e10. doi: 10.1016/j.devcel.2020.10.006. Dev Cell. 2020. PMID: 33171110 Free PMC article.
-
Genome wide assessment of mRNA in astrocyte protrusions by direct RNA sequencing reveals mRNA localization for the intermediate filament protein nestin.Glia. 2013 Nov;61(11):1922-37. doi: 10.1002/glia.22569. Epub 2013 Sep 5. Glia. 2013. PMID: 24009167
-
Sending messages in moving cells: mRNA localization and the regulation of cell migration.Essays Biochem. 2019 Oct 31;63(5):595-606. doi: 10.1042/EBC20190009. Essays Biochem. 2019. PMID: 31324705 Review.
-
The coupled and uncoupled mechanisms by which trans-acting factors regulate mRNA stability and translation.J Biochem. 2017 Apr 1;161(4):309-314. doi: 10.1093/jb/mvw086. J Biochem. 2017. PMID: 28039391 Review.
Cited by
-
Intracellular mRNA transport and localized translation.Nat Rev Mol Cell Biol. 2021 Jul;22(7):483-504. doi: 10.1038/s41580-021-00356-8. Epub 2021 Apr 9. Nat Rev Mol Cell Biol. 2021. PMID: 33837370 Free PMC article. Review.
-
RNA localization in confined cells depends on cellular mechanical activity and contributes to confined migration.iScience. 2022 Feb 1;25(2):103845. doi: 10.1016/j.isci.2022.103845. eCollection 2022 Feb 18. iScience. 2022. PMID: 35198898 Free PMC article.
-
Folylpolyglutamate synthetase mRNA G-quadruplexes regulate its cell protrusion localization and enhance a cancer cell invasive phenotype upon folate repletion.BMC Biol. 2023 Feb 1;21(1):13. doi: 10.1186/s12915-023-01525-1. BMC Biol. 2023. PMID: 36721160 Free PMC article.
-
Where, When, and How: Context-Dependent Functions of RNA Methylation Writers, Readers, and Erasers.Mol Cell. 2019 May 16;74(4):640-650. doi: 10.1016/j.molcel.2019.04.025. Mol Cell. 2019. PMID: 31100245 Free PMC article. Review.
-
Nanoparticle-based local translation reveals mRNA as a translation-coupled scaffold with anchoring function.Proc Natl Acad Sci U S A. 2019 Jul 2;116(27):13346-13351. doi: 10.1073/pnas.1900310116. Epub 2019 Jun 19. Proc Natl Acad Sci U S A. 2019. PMID: 31217293 Free PMC article.
References
-
- Cain R.J., Ridley A.J. Phosphoinositide 3-kinases in cell migration. Biol. Cell. 2009;101:13–29. - PubMed
Publication types
MeSH terms
Substances
Grants and funding
LinkOut - more resources
Full Text Sources
Other Literature Sources
Molecular Biology Databases