Extracellular rigidity sensing by talin isoform-specific mechanical linkages
- PMID: 26523364
- PMCID: PMC4662888
- DOI: 10.1038/ncb3268
Extracellular rigidity sensing by talin isoform-specific mechanical linkages
Abstract
The ability of cells to adhere and sense differences in tissue stiffness is crucial for organ development and function. The central mechanisms by which adherent cells detect extracellular matrix compliance, however, are still unknown. Using two single-molecule-calibrated biosensors that allow the analysis of a previously inaccessible but physiologically highly relevant force regime in cells, we demonstrate that the integrin activator talin establishes mechanical linkages following cell adhesion, which are indispensable for cells to probe tissue stiffness. Talin linkages are exposed to a range of piconewton forces and bear, on average, 7-10 pN during cell adhesion depending on their association with F-actin and vinculin. Disruption of talin's mechanical engagement does not impair integrin activation and initial cell adhesion but prevents focal adhesion reinforcement and thus extracellular rigidity sensing. Intriguingly, talin mechanics are isoform specific so that expression of either talin-1 or talin-2 modulates extracellular rigidity sensing.
Figures
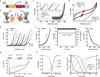
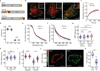
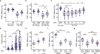
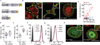
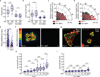
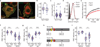
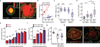
Comment in
-
Sensing some tension.Nat Methods. 2016 Jan;13(1):17. doi: 10.1038/nmeth.3726. Nat Methods. 2016. PMID: 27110628 No abstract available.
Similar articles
-
How vinculin regulates force transmission.Proc Natl Acad Sci U S A. 2013 Jun 11;110(24):9788-93. doi: 10.1073/pnas.1216209110. Epub 2013 May 28. Proc Natl Acad Sci U S A. 2013. PMID: 23716647 Free PMC article.
-
A small proportion of Talin molecules transmit forces at developing muscle attachments in vivo.PLoS Biol. 2019 Mar 27;17(3):e3000057. doi: 10.1371/journal.pbio.3000057. eCollection 2019 Mar. PLoS Biol. 2019. PMID: 30917109 Free PMC article.
-
Vinculin controls talin engagement with the actomyosin machinery.Nat Commun. 2015 Dec 4;6:10038. doi: 10.1038/ncomms10038. Nat Commun. 2015. PMID: 26634421 Free PMC article.
-
Integrating actin dynamics, mechanotransduction and integrin activation: the multiple functions of actin binding proteins in focal adhesions.Eur J Cell Biol. 2013 Oct-Nov;92(10-11):339-48. doi: 10.1016/j.ejcb.2013.10.009. Epub 2013 Nov 4. Eur J Cell Biol. 2013. PMID: 24252517 Review.
-
Molecular mechanisms underlying the force-dependent regulation of actin-to-ECM linkage at the focal adhesions.Prog Mol Biol Transl Sci. 2014;126:135-54. doi: 10.1016/B978-0-12-394624-9.00006-3. Prog Mol Biol Transl Sci. 2014. PMID: 25081617 Review.
Cited by
-
Techniques to stimulate and interrogate cell-cell adhesion mechanics.Extreme Mech Lett. 2018 Apr;20:125-139. doi: 10.1016/j.eml.2017.12.002. Epub 2017 Dec 7. Extreme Mech Lett. 2018. PMID: 30320194 Free PMC article.
-
Novel roles for scleraxis in regulating adult tenocyte function.BMC Cell Biol. 2018 Aug 7;19(1):14. doi: 10.1186/s12860-018-0166-z. BMC Cell Biol. 2018. PMID: 30086712 Free PMC article.
-
Multitier mechanics control stromal adaptations in the swelling lymph node.Nat Immunol. 2022 Aug;23(8):1246-1255. doi: 10.1038/s41590-022-01257-4. Epub 2022 Jul 11. Nat Immunol. 2022. PMID: 35817845 Free PMC article.
-
Curved adhesions mediate cell attachment to soft matrix fibres in three dimensions.Nat Cell Biol. 2023 Oct;25(10):1453-1464. doi: 10.1038/s41556-023-01238-1. Epub 2023 Sep 28. Nat Cell Biol. 2023. PMID: 37770566 Free PMC article.
-
Associations of carotid intima media thickness with gene expression in whole blood and genetically predicted gene expression across 48 tissues.Hum Mol Genet. 2022 Mar 31;31(7):1171-1182. doi: 10.1093/hmg/ddab236. Hum Mol Genet. 2022. PMID: 34788810 Free PMC article.
References
-
- Heisenberg CP, Bellaiche Y. Forces in tissue morphogenesis and patterning. Cell. 2013;153:948–962. - PubMed
-
- Franze K, Janmey PA, Guck J. Mechanics in neuronal development and repair. Annu. Rev. Biomed. Eng. 2013;15:227–251. - PubMed
-
- Ingber DE. Mechanobiology and diseases of mechanotransduction. Ann. Med. 2003;35:564–577. - PubMed
Publication types
MeSH terms
Substances
Grants and funding
LinkOut - more resources
Full Text Sources
Other Literature Sources
Molecular Biology Databases
Research Materials