In-depth study of Mollivirus sibericum, a new 30,000-y-old giant virus infecting Acanthamoeba
- PMID: 26351664
- PMCID: PMC4586845
- DOI: 10.1073/pnas.1510795112
In-depth study of Mollivirus sibericum, a new 30,000-y-old giant virus infecting Acanthamoeba
Abstract
Acanthamoeba species are infected by the largest known DNA viruses. These include icosahedral Mimiviruses, amphora-shaped Pandoraviruses, and Pithovirus sibericum, the latter one isolated from 30,000-y-old permafrost. Mollivirus sibericum, a fourth type of giant virus, was isolated from the same permafrost sample. Its approximately spherical virion (0.6-µm diameter) encloses a 651-kb GC-rich genome encoding 523 proteins of which 64% are ORFans; 16% have their closest homolog in Pandoraviruses and 10% in Acanthamoeba castellanii probably through horizontal gene transfer. The Mollivirus nucleocytoplasmic replication cycle was analyzed using a combination of "omic" approaches that revealed how the virus highjacks its host machinery to actively replicate. Surprisingly, the host's ribosomal proteins are packaged in the virion. Metagenomic analysis of the permafrost sample uncovered the presence of both viruses, yet in very low amount. The fact that two different viruses retain their infectivity in prehistorical permafrost layers should be of concern in a context of global warming. Giant viruses' diversity remains to be fully explored.
Keywords: Pleistocene; giant virus; permafrost.
Conflict of interest statement
The authors declare no conflict of interest.
Figures
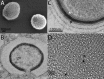
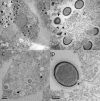
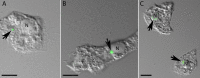
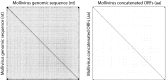
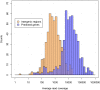
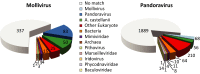
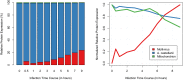
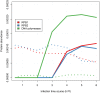
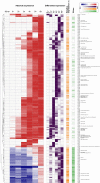
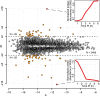
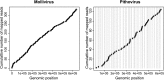
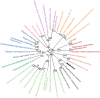
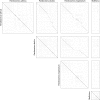
Similar articles
-
Characterization of Mollivirus kamchatka, the First Modern Representative of the Proposed Molliviridae Family of Giant Viruses.J Virol. 2020 Mar 31;94(8):e01997-19. doi: 10.1128/JVI.01997-19. Print 2020 Mar 31. J Virol. 2020. PMID: 31996429 Free PMC article.
-
Complex Membrane Remodeling during Virion Assembly of the 30,000-Year-Old Mollivirus Sibericum.J Virol. 2019 Jun 14;93(13):e00388-19. doi: 10.1128/JVI.00388-19. Print 2019 Jul 1. J Virol. 2019. PMID: 30996095 Free PMC article.
-
Thirty-thousand-year-old distant relative of giant icosahedral DNA viruses with a pandoravirus morphology.Proc Natl Acad Sci U S A. 2014 Mar 18;111(11):4274-9. doi: 10.1073/pnas.1320670111. Epub 2014 Mar 3. Proc Natl Acad Sci U S A. 2014. PMID: 24591590 Free PMC article.
-
Sputnik, a virophage infecting the viral domain of life.Adv Virus Res. 2012;82:63-89. doi: 10.1016/B978-0-12-394621-8.00013-3. Adv Virus Res. 2012. PMID: 22420851 Review.
-
Giant Viruses of Amoebas: An Update.Front Microbiol. 2016 Mar 22;7:349. doi: 10.3389/fmicb.2016.00349. eCollection 2016. Front Microbiol. 2016. PMID: 27047465 Free PMC article. Review.
Cited by
-
Quantitative conversion of biomass in giant DNA virus infection.Sci Rep. 2021 Mar 3;11(1):5025. doi: 10.1038/s41598-021-83547-9. Sci Rep. 2021. PMID: 33658544 Free PMC article.
-
Kaumoebavirus, a New Virus That Clusters with Faustoviruses and Asfarviridae.Viruses. 2016 Oct 28;8(11):278. doi: 10.3390/v8110278. Viruses. 2016. PMID: 27801826 Free PMC article.
-
On the occurrence of cytochrome P450 in viruses.Proc Natl Acad Sci U S A. 2019 Jun 18;116(25):12343-12352. doi: 10.1073/pnas.1901080116. Epub 2019 Jun 5. Proc Natl Acad Sci U S A. 2019. PMID: 31167942 Free PMC article.
-
Taxon Richness of "Megaviridae" Exceeds those of Bacteria and Archaea in the Ocean.Microbes Environ. 2018 Jul 4;33(2):162-171. doi: 10.1264/jsme2.ME17203. Epub 2018 May 25. Microbes Environ. 2018. PMID: 29806626 Free PMC article.
-
The Large Marseillevirus Explores Different Entry Pathways by Forming Giant Infectious Vesicles.J Virol. 2016 May 12;90(11):5246-55. doi: 10.1128/JVI.00177-16. Print 2016 Jun 1. J Virol. 2016. PMID: 26984730 Free PMC article.
References
Publication types
MeSH terms
Substances
Associated data
LinkOut - more resources
Full Text Sources
Other Literature Sources
Miscellaneous