The Daxx/Atrx Complex Protects Tandem Repetitive Elements during DNA Hypomethylation by Promoting H3K9 Trimethylation
- PMID: 26340527
- PMCID: PMC4571182
- DOI: 10.1016/j.stem.2015.07.022
The Daxx/Atrx Complex Protects Tandem Repetitive Elements during DNA Hypomethylation by Promoting H3K9 Trimethylation
Abstract
In mammals, DNA methylation is essential for protecting repetitive sequences from aberrant transcription and recombination. In some developmental contexts (e.g., preimplantation embryos) DNA is hypomethylated but repetitive elements are not dysregulated, suggesting that alternative protection mechanisms exist. Here we explore the processes involved by investigating the role of the chromatin factors Daxx and Atrx. Using genome-wide binding and transcriptome analysis, we found that Daxx and Atrx have distinct chromatin-binding profiles and are co-enriched at tandem repetitive elements in wild-type mouse ESCs. Global DNA hypomethylation further promoted recruitment of the Daxx/Atrx complex to tandem repeat sequences, including retrotransposons and telomeres. Knockdown of Daxx/Atrx in cells with hypomethylated genomes exacerbated aberrant transcriptional de-repression of repeat elements and telomere dysfunction. Mechanistically, Daxx/Atrx-mediated repression seems to involve Suv39h recruitment and H3K9 trimethylation. Our data therefore suggest that Daxx and Atrx safeguard the genome by silencing repetitive elements when DNA methylation levels are low.
Keywords: ATRX; DAXX; DNA methylation; DNMTs; histone modification; mouse embryonic stem cells; repetitive sequences; telomeres.
Copyright © 2015 Elsevier Inc. All rights reserved.
Conflict of interest statement
The authors declare no competing financial interests.
Figures
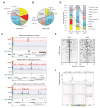
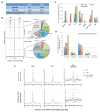
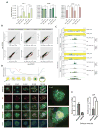
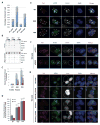
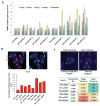
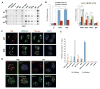
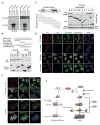
Comment in
-
Adaption by Rewiring Epigenetic Landscapes.Cell Stem Cell. 2015 Sep 3;17(3):249-50. doi: 10.1016/j.stem.2015.08.015. Cell Stem Cell. 2015. PMID: 26340521 Free PMC article.
Similar articles
-
Adaption by Rewiring Epigenetic Landscapes.Cell Stem Cell. 2015 Sep 3;17(3):249-50. doi: 10.1016/j.stem.2015.08.015. Cell Stem Cell. 2015. PMID: 26340521 Free PMC article.
-
Daxx is an H3.3-specific histone chaperone and cooperates with ATRX in replication-independent chromatin assembly at telomeres.Proc Natl Acad Sci U S A. 2010 Aug 10;107(32):14075-80. doi: 10.1073/pnas.1008850107. Epub 2010 Jul 22. Proc Natl Acad Sci U S A. 2010. PMID: 20651253 Free PMC article.
-
Single-cell analysis of Daxx and ATRX-dependent transcriptional repression.J Cell Sci. 2012 Nov 15;125(Pt 22):5489-501. doi: 10.1242/jcs.110148. Epub 2012 Sep 12. J Cell Sci. 2012. PMID: 22976303 Free PMC article.
-
ATRX and DAXX: Mechanisms and Mutations.Cold Spring Harb Perspect Med. 2017 Mar 1;7(3):a026567. doi: 10.1101/cshperspect.a026567. Cold Spring Harb Perspect Med. 2017. PMID: 28062559 Free PMC article. Review.
-
Emerging roles of ATRX in cancer.Epigenomics. 2015;7(8):1365-78. doi: 10.2217/epi.15.82. Epub 2015 Dec 8. Epigenomics. 2015. PMID: 26646632 Review.
Cited by
-
Histone Chaperones as Cardinal Players in Development.Front Cell Dev Biol. 2022 Apr 4;10:767773. doi: 10.3389/fcell.2022.767773. eCollection 2022. Front Cell Dev Biol. 2022. PMID: 35445016 Free PMC article. Review.
-
ATRX loss induces telomere dysfunction and necessitates induction of alternative lengthening of telomeres during human cell immortalization.EMBO J. 2019 Oct 1;38(19):e96659. doi: 10.15252/embj.201796659. Epub 2019 Aug 27. EMBO J. 2019. PMID: 31454099 Free PMC article.
-
Loss of Nexmif results in the expression of phenotypic variability and loss of genomic integrity.Sci Rep. 2022 Aug 15;12(1):13815. doi: 10.1038/s41598-022-17845-1. Sci Rep. 2022. PMID: 35970867 Free PMC article.
-
Role of Transposable Elements in Genome Stability: Implications for Health and Disease.Int J Mol Sci. 2022 Jul 15;23(14):7802. doi: 10.3390/ijms23147802. Int J Mol Sci. 2022. PMID: 35887150 Free PMC article. Review.
-
G-quadruplex DNA drives genomic instability and represents a targetable molecular abnormality in ATRX-deficient malignant glioma.Nat Commun. 2019 Feb 26;10(1):943. doi: 10.1038/s41467-019-08905-8. Nat Commun. 2019. PMID: 30808951 Free PMC article.
References
-
- Armour JA, Anttinen T, May CA, Vega EE, Sajantila A, Kidd JR, Kidd KK, Bertranpetit J, Paabo S, Jeffreys AJ. Minisatellite diversity supports a recent African origin for modern humans. Nat Genet. 1996;13:154–160. - PubMed
-
- Azzalin CM, Reichenbach P, Khoriauli L, Giulotto E, Lingner J. Telomeric repeat containing RNA and RNA surveillance factors at mammalian chromosome ends. Science. 2007;318:798–801. - PubMed
Publication types
MeSH terms
Substances
Associated data
- Actions
Grants and funding
LinkOut - more resources
Full Text Sources
Other Literature Sources