Cloak and Dagger: Alternative Immune Evasion and Modulation Strategies of Poxviruses
- PMID: 26308043
- PMCID: PMC4576205
- DOI: 10.3390/v7082844
Cloak and Dagger: Alternative Immune Evasion and Modulation Strategies of Poxviruses
Abstract
As all viruses rely on cellular factors throughout their replication cycle, to be successful they must evolve strategies to evade and/or manipulate the defence mechanisms employed by the host cell. In addition to their expression of a wide array of host modulatory factors, several recent studies have suggested that poxviruses may have evolved unique mechanisms to shunt or evade host detection. These potential mechanisms include mimicry of apoptotic bodies by mature virions (MVs), the use of viral sub-structures termed lateral bodies for the packaging and delivery of host modulators, and the formation of a second, "cloaked" form of infectious extracellular virus (EVs). Here we discuss these various strategies and how they may facilitate poxvirus immune evasion. Finally we propose a model for the exploitation of the cellular exosome pathway for the formation of EVs.
Keywords: exocytosis; exosome; immune evasion; vaccinia virus; virus entry.
Figures
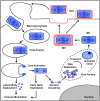
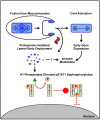
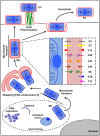
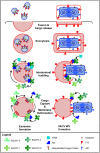
Similar articles
-
Poxvirus Immune Evasion.Annu Rev Immunol. 2024 Jun;42(1):551-584. doi: 10.1146/annurev-immunol-090222-110227. Annu Rev Immunol. 2024. PMID: 38941604 Review.
-
Poxviruses Utilize Multiple Strategies to Inhibit Apoptosis.Viruses. 2017 Aug 8;9(8):215. doi: 10.3390/v9080215. Viruses. 2017. PMID: 28786952 Free PMC article. Review.
-
Poxvirus host cell entry.Curr Opin Virol. 2012 Feb;2(1):20-7. doi: 10.1016/j.coviro.2011.11.007. Epub 2011 Dec 27. Curr Opin Virol. 2012. PMID: 22440962 Review.
-
How vaccinia virus has evolved to subvert the host immune response.J Struct Biol. 2011 Aug;175(2):127-34. doi: 10.1016/j.jsb.2011.03.010. Epub 2011 Mar 17. J Struct Biol. 2011. PMID: 21419849 Free PMC article. Review.
-
Stress Beyond Translation: Poxviruses and More.Viruses. 2016 Jun 14;8(6):169. doi: 10.3390/v8060169. Viruses. 2016. PMID: 27314378 Free PMC article. Review.
Cited by
-
Challenges and Achievements in Prevention and Treatment of Smallpox.Vaccines (Basel). 2018 Jan 29;6(1):8. doi: 10.3390/vaccines6010008. Vaccines (Basel). 2018. PMID: 29382130 Free PMC article. Review.
-
Poxviruses package viral redox proteins in lateral bodies and modulate the host oxidative response.PLoS Pathog. 2022 Jul 14;18(7):e1010614. doi: 10.1371/journal.ppat.1010614. eCollection 2022 Jul. PLoS Pathog. 2022. PMID: 35834477 Free PMC article.
-
Vaccinia virus hijacks EGFR signalling to enhance virus spread through rapid and directed infected cell motility.Nat Microbiol. 2019 Feb;4(2):216-225. doi: 10.1038/s41564-018-0288-2. Epub 2018 Nov 12. Nat Microbiol. 2019. PMID: 30420785 Free PMC article.
-
Vaccinia virus subverts xenophagy through phosphorylation and nuclear targeting of p62.J Cell Biol. 2024 Jun 3;223(6):e202104129. doi: 10.1083/jcb.202104129. Epub 2024 May 6. J Cell Biol. 2024. PMID: 38709216 Free PMC article.
-
ISG15 Is Required for the Dissemination of Vaccinia Virus Extracellular Virions.Microbiol Spectr. 2023 Jun 15;11(3):e0450822. doi: 10.1128/spectrum.04508-22. Epub 2023 Apr 10. Microbiol Spectr. 2023. PMID: 37036376 Free PMC article.
References
Publication types
MeSH terms
Grants and funding
LinkOut - more resources
Full Text Sources
Other Literature Sources
Research Materials