Ubp6 deubiquitinase controls conformational dynamics and substrate degradation of the 26S proteasome
- PMID: 26301997
- PMCID: PMC4560640
- DOI: 10.1038/nsmb.3075
Ubp6 deubiquitinase controls conformational dynamics and substrate degradation of the 26S proteasome
Abstract
Substrates are targeted for proteasomal degradation through the attachment of ubiquitin chains that need to be removed by proteasomal deubiquitinases before substrate processing. In budding yeast, the deubiquitinase Ubp6 trims ubiquitin chains and affects substrate processing by the proteasome, but the underlying mechanisms and the location of Ubp6 within the holoenzyme have been elusive. Here we show that Ubp6 activity strongly responds to interactions with the base ATPase and the conformational state of the proteasome. Electron microscopy analyses reveal that ubiquitin-bound Ubp6 contacts the N ring and AAA+ ring of the ATPase hexamer and is in proximity to the deubiquitinase Rpn11. Ubiquitin-bound Ubp6 inhibits substrate deubiquitination by Rpn11, stabilizes the substrate-engaged conformation of the proteasome and allosterically interferes with the engagement of a subsequent substrate. Ubp6 may thus act as a ubiquitin-dependent 'timer' to coordinate individual processing steps at the proteasome and modulate substrate degradation.
Figures
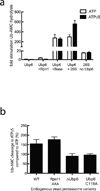
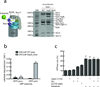
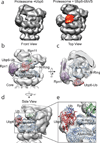
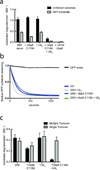
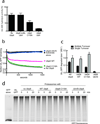
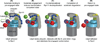
Comment in
-
A timer to coordinate substrate processing by the 26S proteasome.Nat Struct Mol Biol. 2015 Sep;22(9):652-3. doi: 10.1038/nsmb.3085. Nat Struct Mol Biol. 2015. PMID: 26333712 No abstract available.
Similar articles
-
Structural characterization of the interaction of Ubp6 with the 26S proteasome.Proc Natl Acad Sci U S A. 2015 Jul 14;112(28):8626-31. doi: 10.1073/pnas.1510449112. Epub 2015 Jun 30. Proc Natl Acad Sci U S A. 2015. PMID: 26130806 Free PMC article.
-
An AAA Motor-Driven Mechanical Switch in Rpn11 Controls Deubiquitination at the 26S Proteasome.Mol Cell. 2017 Sep 7;67(5):799-811.e8. doi: 10.1016/j.molcel.2017.07.023. Epub 2017 Aug 24. Mol Cell. 2017. PMID: 28844860
-
Deubiquitinating enzyme Ubp6 functions noncatalytically to delay proteasomal degradation.Cell. 2006 Oct 6;127(1):99-111. doi: 10.1016/j.cell.2006.07.038. Cell. 2006. PMID: 17018280
-
Structure, Dynamics and Function of the 26S Proteasome.Subcell Biochem. 2021;96:1-151. doi: 10.1007/978-3-030-58971-4_1. Subcell Biochem. 2021. PMID: 33252727 Review.
-
Deubiquitinating enzymes are IN/(trinsic to proteasome function).Curr Protein Pept Sci. 2004 Jun;5(3):201-11. doi: 10.2174/1389203043379756. Curr Protein Pept Sci. 2004. PMID: 15188770 Review.
Cited by
-
Ubiquitin-dependent switch during assembly of the proteasomal ATPases mediated by Not4 ubiquitin ligase.Proc Natl Acad Sci U S A. 2018 Dec 26;115(52):13246-13251. doi: 10.1073/pnas.1805353115. Epub 2018 Dec 10. Proc Natl Acad Sci U S A. 2018. PMID: 30530678 Free PMC article.
-
How the 26S Proteasome Degrades Ubiquitinated Proteins in the Cell.Biomolecules. 2019 Aug 22;9(9):395. doi: 10.3390/biom9090395. Biomolecules. 2019. PMID: 31443414 Free PMC article. Review.
-
SAXS/MC studies of the mixed-folded protein Cdt1 reveal monomeric, folded over conformations.bioRxiv [Preprint]. 2024 Jan 3:2024.01.03.573975. doi: 10.1101/2024.01.03.573975. bioRxiv. 2024. Update in: Cytoskeleton (Hoboken). 2024 Nov 6. doi: 10.1002/cm.21954 PMID: 38260441 Free PMC article. Updated. Preprint.
-
Mechanisms That Activate 26S Proteasomes and Enhance Protein Degradation.Biomolecules. 2021 May 22;11(6):779. doi: 10.3390/biom11060779. Biomolecules. 2021. PMID: 34067263 Free PMC article. Review.
-
An atomic structure of the human 26S proteasome.Nat Struct Mol Biol. 2016 Sep;23(9):778-85. doi: 10.1038/nsmb.3273. Epub 2016 Jul 18. Nat Struct Mol Biol. 2016. PMID: 27428775
References
-
- Goldberg AL. Protein degradation and protection against misfolded or damaged proteins. Nature. 2003;426:895–899. - PubMed
-
- Goldberg AL. Functions of the proteasome: from protein degradation and immune surveillance to cancer therapy. Biochem. Soc. Trans. 2007;35:12–17. - PubMed
-
- Verma R, et al. Role of Rpn11 metalloprotease in deubiquitination and degradation by the 26S proteasome. Science. 2002;298:611–615. - PubMed
-
- Yao T, Cohen RE. A cryptic protease couples deubiquitination and degradation by the proteasome. Nature. 2002;419:403–407. - PubMed
Publication types
MeSH terms
Substances
Grants and funding
LinkOut - more resources
Full Text Sources
Other Literature Sources
Molecular Biology Databases
Research Materials