MEG3 long noncoding RNA regulates the TGF-β pathway genes through formation of RNA-DNA triplex structures
- PMID: 26205790
- PMCID: PMC4525211
- DOI: 10.1038/ncomms8743
MEG3 long noncoding RNA regulates the TGF-β pathway genes through formation of RNA-DNA triplex structures
Erratum in
-
Author Correction: MEG3 long noncoding RNA regulates the TGF-β pathway genes through formation of RNA-DNA triplex structures.Nat Commun. 2019 Nov 21;10(1):5290. doi: 10.1038/s41467-019-13200-7. Nat Commun. 2019. PMID: 31754097 Free PMC article.
Abstract
Long noncoding RNAs (lncRNAs) regulate gene expression by association with chromatin, but how they target chromatin remains poorly understood. We have used chromatin RNA immunoprecipitation-coupled high-throughput sequencing to identify 276 lncRNAs enriched in repressive chromatin from breast cancer cells. Using one of the chromatin-interacting lncRNAs, MEG3, we explore the mechanisms by which lncRNAs target chromatin. Here we show that MEG3 and EZH2 share common target genes, including the TGF-β pathway genes. Genome-wide mapping of MEG3 binding sites reveals that MEG3 modulates the activity of TGF-β genes by binding to distal regulatory elements. MEG3 binding sites have GA-rich sequences, which guide MEG3 to the chromatin through RNA-DNA triplex formation. We have found that RNA-DNA triplex structures are widespread and are present over the MEG3 binding sites associated with the TGF-β pathway genes. Our findings suggest that RNA-DNA triplex formation could be a general characteristic of target gene recognition by the chromatin-interacting lncRNAs.
Figures
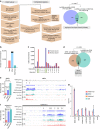
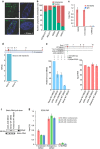
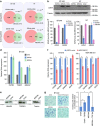
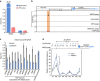
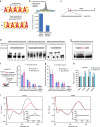
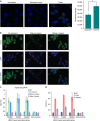
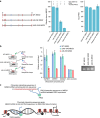
Similar articles
-
MEG3 Long Noncoding RNA Contributes to the Epigenetic Regulation of Epithelial-Mesenchymal Transition in Lung Cancer Cell Lines.J Biol Chem. 2017 Jan 6;292(1):82-99. doi: 10.1074/jbc.M116.750950. Epub 2016 Nov 16. J Biol Chem. 2017. PMID: 27852821 Free PMC article.
-
Long Noncoding RNA Meg3 Regulates Mafa Expression in Mouse Beta Cells by Inactivating Rad21, Smc3 or Sin3α.Cell Physiol Biochem. 2018;45(5):2031-2043. doi: 10.1159/000487983. Epub 2018 Mar 6. Cell Physiol Biochem. 2018. PMID: 29529600
-
Long Noncoding RNA MEG3 Is an Epigenetic Determinant of Oncogenic Signaling in Functional Pancreatic Neuroendocrine Tumor Cells.Mol Cell Biol. 2017 Oct 27;37(22):e00278-17. doi: 10.1128/MCB.00278-17. Print 2017 Nov 15. Mol Cell Biol. 2017. PMID: 28847847 Free PMC article.
-
RNA-DNA Triplex Formation by Long Noncoding RNAs.Cell Chem Biol. 2016 Nov 17;23(11):1325-1333. doi: 10.1016/j.chembiol.2016.09.011. Epub 2016 Oct 20. Cell Chem Biol. 2016. PMID: 27773629 Review.
-
Long noncoding RNAs: Novel insights into hepatocelluar carcinoma.Cancer Lett. 2014 Mar 1;344(1):20-27. doi: 10.1016/j.canlet.2013.10.021. Epub 2013 Oct 30. Cancer Lett. 2014. PMID: 24183851 Review.
Cited by
-
KCNQ1OT1 promotes genome-wide transposon repression by guiding RNA-DNA triplexes and HP1 binding.Nat Cell Biol. 2022 Nov;24(11):1617-1629. doi: 10.1038/s41556-022-01008-5. Epub 2022 Oct 20. Nat Cell Biol. 2022. PMID: 36266489
-
A universal model of RNA.DNA:DNA triplex formation accurately predicts genome-wide RNA-DNA interactions.Brief Bioinform. 2022 Nov 19;23(6):bbac445. doi: 10.1093/bib/bbac445. Brief Bioinform. 2022. PMID: 36239395 Free PMC article.
-
An Overview of the Immune Modulatory Properties of Long Non-Coding RNAs and Their Potential Use as Therapeutic Targets in Cancer.Noncoding RNA. 2023 Nov 11;9(6):70. doi: 10.3390/ncrna9060070. Noncoding RNA. 2023. PMID: 37987366 Free PMC article. Review.
-
Transcription regulation by long non-coding RNAs: mechanisms and disease relevance.Nat Rev Mol Cell Biol. 2024 May;25(5):396-415. doi: 10.1038/s41580-023-00694-9. Epub 2024 Jan 19. Nat Rev Mol Cell Biol. 2024. PMID: 38242953 Free PMC article. Review.
-
Functional impact of the long non-coding RNA MEG3 deletion by CRISPR/Cas9 in the human triple negative metastatic Hs578T cancer cell line.Oncol Lett. 2019 Dec;18(6):5941-5951. doi: 10.3892/ol.2019.10969. Epub 2019 Oct 8. Oncol Lett. 2019. PMID: 31788068 Free PMC article.
References
-
- Mohammad F., Mondal T., Guseva N., Pandey G. K. & Kanduri C. Kcnq1ot1 noncoding RNA mediates transcriptional gene silencing by interacting with Dnmt1. Development 137, 2493–2499 (2010). - PubMed
Publication types
MeSH terms
Substances
LinkOut - more resources
Full Text Sources
Other Literature Sources
Research Materials