μ opioid receptor activation hyperpolarizes respiratory-controlling Kölliker-Fuse neurons and suppresses post-inspiratory drive
- PMID: 26175072
- PMCID: PMC4594241
- DOI: 10.1113/JP270822
μ opioid receptor activation hyperpolarizes respiratory-controlling Kölliker-Fuse neurons and suppresses post-inspiratory drive
Abstract
Key points: In addition to reductions in respiratory rate, opioids also cause aspiration and difficulty swallowing, indicating impairment of the upper airways. The Kölliker-Fuse (KF) maintains upper airway patency and a normal respiratory pattern. In this study, activation of μ opioid receptors in the KF reduced respiratory frequency and tidal volume in anaesthetized rats. Nerve recordings in an in situ preparation showed that activation of μ opioid receptors in the KF eliminated the post-inspiration phase of the respiratory cycle. In brain slices, μ opioid agonists hyperpolarized a distinct population (61%) of KF neurons by activation of an inwardly rectifying potassium conductance. These results suggest that KF neurons that are hyperpolarized by opioids could contribute to opioid-induced respiratory disturbances, particularly the impairment of upper airways.
Abstract: Opioid-induced respiratory effects include aspiration and difficulty swallowing, suggesting impairment of the upper airways. The pontine Kölliker-Fuse nucleus (KF) controls upper airway patency and regulates respiration, in particular the inspiratory/expiratory phase transition. Given the importance of the KF in coordinating respiratory pattern, the mechanisms of μ opioid receptor activation in this nucleus were investigated at the systems and cellular level. In anaesthetized, vagi-intact rats, injection of opioid agonists DAMGO or [Met(5) ]enkephalin (ME) into the KF reduced respiratory frequency and amplitude. The μ opioid agonist DAMGO applied directly into the KF of the in situ arterially perfused working heart-brainstem preparation of rat resulted in robust apneusis (lengthened low amplitude inspiration due to loss of post-inspiratory drive) that was rapidly reversed by the opioid antagonist naloxone. In brain slice preparations, activation of μ opioid receptors on KF neurons hyperpolarized a distinct population (61%) of neurons. As expected, the opioid-induced hyperpolarization reduced the excitability of the neuron in response to either current injection or local application of glutamate. In voltage-clamp recordings the outward current produced by the opioid agonist ME was concentration dependent, reversed at the potassium equilibrium potential and was blocked by BaCl2 , characteristics of a G protein-coupled inwardly rectifying potassium (GIRK) conductance. The clinically used drug morphine produced an outward current in KF neurons with similar potency to morphine-mediated currents in locus coeruleus brain slice preparations. Thus, the population of KF neurons that are hyperpolarized by μ opioid agonists are likely mediators of the opioid-induced loss of post-inspiration and induction of apneusis.
© 2015 The Authors. The Journal of Physiology © 2015 The Physiological Society.
Figures
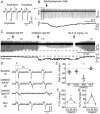

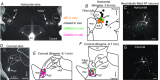
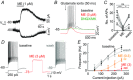
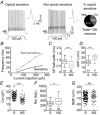
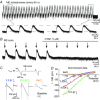
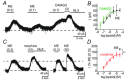
Similar articles
-
Desensitization and Tolerance of Mu Opioid Receptors on Pontine Kölliker-Fuse Neurons.Mol Pharmacol. 2018 Jan;93(1):8-13. doi: 10.1124/mol.117.109603. Epub 2017 Nov 2. Mol Pharmacol. 2018. PMID: 29097440 Free PMC article.
-
Kölliker-Fuse/Parabrachial complex mu opioid receptors contribute to fentanyl-induced apnea and respiratory rate depression.Respir Physiol Neurobiol. 2020 Apr;275:103388. doi: 10.1016/j.resp.2020.103388. Epub 2020 Jan 15. Respir Physiol Neurobiol. 2020. PMID: 31953234 Free PMC article.
-
Mu-opioid-mediated inhibition of glutamate synaptic transmission in rat central amygdala neurons.Neuroscience. 2005;133(1):97-103. doi: 10.1016/j.neuroscience.2005.02.004. Neuroscience. 2005. PMID: 15893634
-
Mechanisms of opioid-induced respiratory depression.Arch Toxicol. 2022 Aug;96(8):2247-2260. doi: 10.1007/s00204-022-03300-7. Epub 2022 Apr 26. Arch Toxicol. 2022. PMID: 35471232 Review.
-
Neurochemistry of the Kölliker-Fuse nucleus from a respiratory perspective.J Neurochem. 2021 Jan;156(1):16-37. doi: 10.1111/jnc.15041. Epub 2020 Jun 10. J Neurochem. 2021. PMID: 32396650 Free PMC article. Review.
Cited by
-
Advances in attenuating opioid-induced respiratory depression: A narrative review.Medicine (Baltimore). 2024 Jul 19;103(29):e38837. doi: 10.1097/MD.0000000000038837. Medicine (Baltimore). 2024. PMID: 39029082 Free PMC article. Review.
-
Mechanism of opioid addiction and its intervention therapy: Focusing on the reward circuitry and mu-opioid receptor.MedComm (2020). 2022 Jun 22;3(3):e148. doi: 10.1002/mco2.148. eCollection 2022 Sep. MedComm (2020). 2022. PMID: 35774845 Free PMC article. Review.
-
Morphine-induced respiratory depression is independent of β-arrestin2 signalling.Br J Pharmacol. 2020 Jul;177(13):2923-2931. doi: 10.1111/bph.15004. Epub 2020 Feb 17. Br J Pharmacol. 2020. PMID: 32052419 Free PMC article.
-
Kölliker-Fuse GABAergic and glutamatergic neurons project to distinct targets.J Comp Neurol. 2017 Jun 1;525(8):1844-1860. doi: 10.1002/cne.24164. Epub 2017 Mar 14. J Comp Neurol. 2017. PMID: 28032634 Free PMC article.
-
Fentanyl effects on respiratory neuron activity in the dorsolateral pons.J Neurophysiol. 2022 Nov 1;128(5):1117-1132. doi: 10.1152/jn.00113.2022. Epub 2022 Oct 5. J Neurophysiol. 2022. PMID: 36197016 Free PMC article.
References
-
- Bautista TG & Dutschmann M (2014. a). Inhibition of the pontine Kölliker‐Fuse nucleus abolishes eupneic inspiratory hypoglossal motor discharge in rat. Neuroscience 267, 22–29. - PubMed
-
- Burke PGR, Abbott SBG, McMullan S, Goodchild AK & Pilowsky PM (2010). Somatostatin selectively ablates post‐inspiratory activity after injection into the Bötzinger complex. Neuroscience 167, 528–539. - PubMed
Publication types
MeSH terms
Substances
Grants and funding
LinkOut - more resources
Full Text Sources
Other Literature Sources
Research Materials
Miscellaneous