Analysis of the Role of the C-Terminal Tail in the Regulation of the Epidermal Growth Factor Receptor
- PMID: 26124280
- PMCID: PMC4525312
- DOI: 10.1128/MCB.00248-15
Analysis of the Role of the C-Terminal Tail in the Regulation of the Epidermal Growth Factor Receptor
Abstract
The ∼230-residue C-terminal tail of the epidermal growth factor receptor (EGFR) is phosphorylated upon activation. We examined whether this phosphorylation is affected by deletions within the tail and whether the two tails in the asymmetric active EGFR dimer are phosphorylated differently. We monitored autophosphorylation in cells using flow cytometry and found that the first ∼80 residues of the tail are inhibitory, as demonstrated previously. The entire ∼80-residue span is important for autoinhibition and needs to be released from both kinases that form the dimer. These results are interpreted in terms of crystal structures of the inactive kinase domain, including two new ones presented here. Deletions in the remaining portion of the tail do not affect autophosphorylation, except for a six-residue segment spanning Tyr 1086 that is critical for activation loop phosphorylation. Phosphorylation of the two tails in the dimer is asymmetric, with the activator tail being phosphorylated somewhat more strongly. Unexpectedly, we found that reconstitution of the transmembrane and cytoplasmic domains of EGFR in vesicles leads to a peculiar phenomenon in which kinase domains appear to be trapped between stacks of lipid bilayers. This artifactual trapping of kinases between membranes enhances an intrinsic functional asymmetry in the two tails in a dimer.
Copyright © 2015, American Society for Microbiology. All Rights Reserved.
Figures
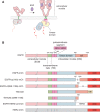
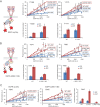
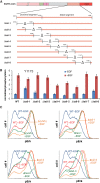
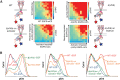
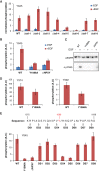
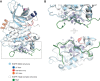
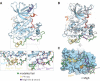
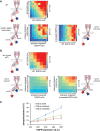
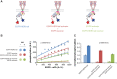
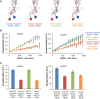
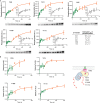
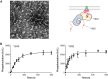
Similar articles
-
An allosteric mechanism for activation of the kinase domain of epidermal growth factor receptor.Cell. 2006 Jun 16;125(6):1137-49. doi: 10.1016/j.cell.2006.05.013. Cell. 2006. PMID: 16777603
-
Modulation of the protein tyrosine kinase activity and autophosphorylation of the epidermal growth factor receptor by its juxtamembrane region.Arch Biochem Biophys. 1999 Mar 15;363(2):227-36. doi: 10.1006/abbi.1998.1095. Arch Biochem Biophys. 1999. PMID: 10068444
-
Conformational regulation of the EGFR kinase core by the juxtamembrane and C-terminal tail: a molecular dynamics study.Proteins. 2011 Jan;79(1):99-114. doi: 10.1002/prot.22862. Epub 2010 Oct 11. Proteins. 2011. PMID: 20938978
-
An electrostatic engine model for autoinhibition and activation of the epidermal growth factor receptor (EGFR/ErbB) family.J Gen Physiol. 2005 Jul;126(1):41-53. doi: 10.1085/jgp.200509274. Epub 2005 Jun 13. J Gen Physiol. 2005. PMID: 15955874 Free PMC article.
-
The ErbB/HER family of protein-tyrosine kinases and cancer.Pharmacol Res. 2014 Jan;79:34-74. doi: 10.1016/j.phrs.2013.11.002. Epub 2013 Nov 20. Pharmacol Res. 2014. PMID: 24269963 Review.
Cited by
-
In-cell structural dynamics of an EGF receptor during ligand-induced dimer-oligomer transition.Eur Biophys J. 2020 Jan;49(1):21-37. doi: 10.1007/s00249-019-01410-2. Epub 2019 Nov 18. Eur Biophys J. 2020. PMID: 31740999
-
The combined action of the intracellular regions regulates FGFR2 kinase activity.Commun Biol. 2023 Jul 14;6(1):728. doi: 10.1038/s42003-023-05112-6. Commun Biol. 2023. PMID: 37452126 Free PMC article.
-
Interdependence between EGFR and Phosphatases Spatially Established by Vesicular Dynamics Generates a Growth Factor Sensing and Responding Network.Cell Syst. 2018 Sep 26;7(3):295-309.e11. doi: 10.1016/j.cels.2018.06.006. Epub 2018 Aug 22. Cell Syst. 2018. PMID: 30145116 Free PMC article.
-
Structural Insight and Development of EGFR Tyrosine Kinase Inhibitors.Molecules. 2022 Jan 26;27(3):819. doi: 10.3390/molecules27030819. Molecules. 2022. PMID: 35164092 Free PMC article. Review.
-
Ligand-induced transmembrane conformational coupling in monomeric EGFR.Nat Commun. 2022 Jul 6;13(1):3709. doi: 10.1038/s41467-022-31299-z. Nat Commun. 2022. PMID: 35794108 Free PMC article.
References
Publication types
MeSH terms
Substances
Associated data
- Actions
- Actions
- Actions
- Actions
Grants and funding
LinkOut - more resources
Full Text Sources
Research Materials
Miscellaneous