Nuclear factor κB-inducing kinase activation as a mechanism of pancreatic β cell failure in obesity
- PMID: 26122662
- PMCID: PMC4516791
- DOI: 10.1084/jem.20150218
Nuclear factor κB-inducing kinase activation as a mechanism of pancreatic β cell failure in obesity
Abstract
The nuclear factor κB (NF-κB) pathway is a master regulator of inflammatory processes and is implicated in insulin resistance and pancreatic β cell dysfunction in the metabolic syndrome. Whereas canonical NF-κB signaling is well studied, there is little information on the divergent noncanonical NF-κB pathway in the context of pancreatic islet dysfunction. Here, we demonstrate that pharmacological activation of the noncanonical NF-κB-inducing kinase (NIK) disrupts glucose homeostasis in zebrafish in vivo. We identify NIK as a critical negative regulator of β cell function, as pharmacological NIK activation results in impaired glucose-stimulated insulin secretion in mouse and human islets. NIK levels are elevated in pancreatic islets isolated from diet-induced obese (DIO) mice, which exhibit increased processing of noncanonical NF-κB components p100 to p52, and accumulation of RelB. TNF and receptor activator of NF-κB ligand (RANKL), two ligands associated with diabetes, induce NIK in islets. Mice with constitutive β cell-intrinsic NIK activation present impaired insulin secretion with DIO. NIK activation triggers the noncanonical NF-κB transcriptional network to induce genes identified in human type 2 diabetes genome-wide association studies linked to β cell failure. These studies reveal that NIK contributes a central mechanism for β cell failure in diet-induced obesity.
© 2015 Malle et al.
Figures
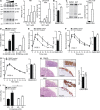
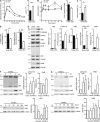
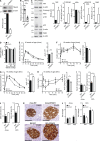
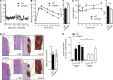
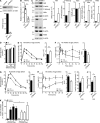
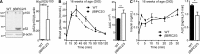
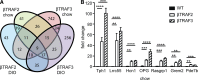
Comment in
-
Saving β cell function in the NIK of time.J Exp Med. 2015 Jul 27;212(8):1140-1. doi: 10.1084/jem.2128insight2. J Exp Med. 2015. PMID: 26216602 Free PMC article. No abstract available.
Similar articles
-
Tumor necrosis factor-like weak inducer of apoptosis (TWEAK) promotes glioma cell invasion through induction of NF-κB-inducing kinase (NIK) and noncanonical NF-κB signaling.Mol Cancer. 2015 Jan 27;14(1):9. doi: 10.1186/s12943-014-0273-1. Mol Cancer. 2015. PMID: 25622756 Free PMC article.
-
RANKL/RANK contributes to the pathological process of type 2 diabetes mellitus through TRAF3 activation of NIK.Int Immunopharmacol. 2024 Dec 5;142(Pt A):113008. doi: 10.1016/j.intimp.2024.113008. Epub 2024 Aug 31. Int Immunopharmacol. 2024. PMID: 39217877
-
NF-κB-inducing kinase (NIK) is activated in pancreatic β-cells but does not contribute to the development of diabetes.Cell Death Dis. 2022 May 19;13(5):476. doi: 10.1038/s41419-022-04931-5. Cell Death Dis. 2022. PMID: 35589698 Free PMC article.
-
The noncanonical NF-κB pathway.Immunol Rev. 2012 Mar;246(1):125-40. doi: 10.1111/j.1600-065X.2011.01088.x. Immunol Rev. 2012. PMID: 22435551 Free PMC article. Review.
-
Non-canonical NF-κB signaling pathway.Cell Res. 2011 Jan;21(1):71-85. doi: 10.1038/cr.2010.177. Epub 2010 Dec 21. Cell Res. 2011. PMID: 21173796 Free PMC article. Review.
Cited by
-
Epigenetic link between Agent Orange exposure and type 2 diabetes in Korean veterans.Front Endocrinol (Lausanne). 2024 Jul 12;15:1375459. doi: 10.3389/fendo.2024.1375459. eCollection 2024. Front Endocrinol (Lausanne). 2024. PMID: 39072272 Free PMC article.
-
The Anti-Inflammatory Effect of Novel Antidiabetic Agents.Life (Basel). 2022 Nov 9;12(11):1829. doi: 10.3390/life12111829. Life (Basel). 2022. PMID: 36362984 Free PMC article. Review.
-
Extra-osseous Roles of the RANK-RANKL-OPG Axis with a Focus on Skeletal Muscle.Curr Osteoporos Rep. 2024 Dec;22(6):632-650. doi: 10.1007/s11914-024-00890-2. Epub 2024 Sep 26. Curr Osteoporos Rep. 2024. PMID: 39325366 Free PMC article. Review.
-
Intracutaneous Transplantation of Islets Within a Biodegradable Temporizing Matrix as an Alternative Site for Islet Transplantation.Diabetes. 2023 Jun 1;72(6):758-768. doi: 10.2337/db21-0841. Diabetes. 2023. PMID: 36929171 Free PMC article.
-
The novel long noncoding RNA lncRNA-Adi regulates adipogenesis.Stem Cells Transl Med. 2020 Sep;9(9):1053-1067. doi: 10.1002/sctm.19-0438. Epub 2020 May 1. Stem Cells Transl Med. 2020. PMID: 32356938 Free PMC article.
References
-
- Ambra R., Manca S., Palumbo M.C., Leoni G., Natarelli L., De Marco A., Consoli A., Pandolfi A., and Virgili F.. 2014. Transcriptome analysis of human primary endothelial cells (HUVEC) from umbilical cords of gestational diabetic mothers reveals candidate sites for an epigenetic modulation of specific gene expression. Genomics. 103:337–348. 10.1016/j.ygeno.2014.03.003 - DOI - PubMed
-
- Ammälä C., Eliasson L., Bokvist K., Berggren P.O., Honkanen R.E., Sjöholm A., and Rorsman P.. 1994. Activation of protein kinases and inhibition of protein phosphatases play a central role in the regulation of exocytosis in mouse pancreatic beta cells. Proc. Natl. Acad. Sci. USA. 91:4343–4347. 10.1073/pnas.91.10.4343 - DOI - PMC - PubMed
-
- Bonizzi G., Bebien M., Otero D.C., Johnson-Vroom K.E., Cao Y., Vu D., Jegga A.G., Aronow B.J., Ghosh G., Rickert R.C., and Karin M.. 2004. Activation of IKKalpha target genes depends on recognition of specific kappaB binding sites by RelB:p52 dimers. EMBO J. 23:4202–4210. 10.1038/sj.emboj.7600391 - DOI - PMC - PubMed
-
- Bradfield J.P., Qu H.Q., Wang K., Zhang H., Sleiman P.M., Kim C.E., Mentch F.D., Qiu H., Glessner J.T., Thomas K.A., et al. . 2011. A genome-wide meta-analysis of six type 1 diabetes cohorts identifies multiple associated loci. PLoS Genet. 7:e1002293 10.1371/journal.pgen.1002293 - DOI - PMC - PubMed
Publication types
MeSH terms
Substances
LinkOut - more resources
Full Text Sources
Other Literature Sources
Medical
Molecular Biology Databases
Miscellaneous