Crystal Structure of Antagonist Bound Human Lysophosphatidic Acid Receptor 1
- PMID: 26091040
- PMCID: PMC4476059
- DOI: 10.1016/j.cell.2015.06.002
Crystal Structure of Antagonist Bound Human Lysophosphatidic Acid Receptor 1
Abstract
Lipid biology continues to emerge as an area of significant therapeutic interest, particularly as the result of an enhanced understanding of the wealth of signaling molecules with diverse physiological properties. This growth in knowledge is epitomized by lysophosphatidic acid (LPA), which functions through interactions with at least six cognate G protein-coupled receptors. Herein, we present three crystal structures of LPA1 in complex with antagonist tool compounds selected and designed through structural and stability analyses. Structural analysis combined with molecular dynamics identified a basis for ligand access to the LPA1 binding pocket from the extracellular space contrasting with the proposed access for the sphingosine 1-phosphate receptor. Characteristics of the LPA1 binding pocket raise the possibility of promiscuous ligand recognition of phosphorylated endocannabinoids. Cell-based assays confirmed this hypothesis, linking the distinct receptor systems through metabolically related ligands with potential functional and therapeutic implications for treatment of disease.
Copyright © 2015 Elsevier Inc. All rights reserved.
Figures
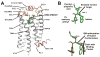
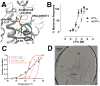
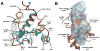
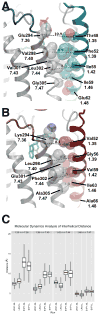
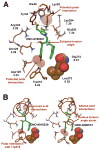
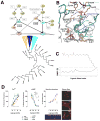
Similar articles
-
Structural insights into ligand recognition by the lysophosphatidic acid receptor LPA6.Nature. 2017 Aug 17;548(7667):356-360. doi: 10.1038/nature23448. Epub 2017 Aug 9. Nature. 2017. PMID: 28792932
-
Sphingosine 1-phosphate and lysophosphatidic acid receptors: agonist and antagonist binding and progress toward development of receptor-specific ligands.Semin Cell Dev Biol. 2004 Oct;15(5):467-76. doi: 10.1016/j.semcdb.2004.05.006. Semin Cell Dev Biol. 2004. PMID: 15271292 Review.
-
Analysis of Edg-Like LPA Receptor-Ligand Interactions.Curr Pharm Des. 2015;21(24):3533-47. doi: 10.2174/1381612821666150216120500. Curr Pharm Des. 2015. PMID: 25686617
-
Crystal structure of a lipid G protein-coupled receptor.Science. 2012 Feb 17;335(6070):851-5. doi: 10.1126/science.1215904. Science. 2012. PMID: 22344443 Free PMC article.
-
Novel clusters of receptors for sphingosine-1-phosphate, sphingosylphosphorylcholine, and (lyso)-phosphatidic acid: new receptors for "old" ligands.J Cell Biochem. 2004 Aug 1;92(5):923-36. doi: 10.1002/jcb.20092. J Cell Biochem. 2004. PMID: 15258916 Review.
Cited by
-
Crystal Structure of the Human Cannabinoid Receptor CB1.Cell. 2016 Oct 20;167(3):750-762.e14. doi: 10.1016/j.cell.2016.10.004. Cell. 2016. PMID: 27768894 Free PMC article.
-
Fingolimod: Lessons Learned and New Opportunities for Treating Multiple Sclerosis and Other Disorders.Annu Rev Pharmacol Toxicol. 2019 Jan 6;59:149-170. doi: 10.1146/annurev-pharmtox-010818-021358. Annu Rev Pharmacol Toxicol. 2019. PMID: 30625282 Free PMC article. Review.
-
CB1 and LPA1 Receptors Relationship in the Mouse Central Nervous System.Front Mol Neurosci. 2019 Sep 19;12:223. doi: 10.3389/fnmol.2019.00223. eCollection 2019. Front Mol Neurosci. 2019. PMID: 31607860 Free PMC article.
-
Recent Advances in Structure, Function, and Pharmacology of Class A Lipid GPCRs: Opportunities and Challenges for Drug Discovery.Pharmaceuticals (Basel). 2021 Dec 22;15(1):12. doi: 10.3390/ph15010012. Pharmaceuticals (Basel). 2021. PMID: 35056070 Free PMC article. Review.
-
Structural and functional insights into the G protein-coupled receptors: CB1 and CB2.Biochem Soc Trans. 2023 Aug 31;51(4):1533-1543. doi: 10.1042/BST20221316. Biochem Soc Trans. 2023. PMID: 37646476 Free PMC article. Review.
References
-
- Alexandrov AI, Mileni M, Chien EY, Hanson MA, Stevens RC. Microscale fluorescent thermal stability assay for membrane proteins. Structure. 2008;16:351–359. - PubMed
-
- Aoki J. Mechanisms of lysophosphatidic acid production. Semin Cell Dev Biol. 2004;15:477–489. - PubMed
-
- Ballesteros JA, Weinstein H. Integrated methods for the construction of three dimensional models and computational probing of structure-function relations in G-protein coupled receptors. Methods Neurosci. 1995;25:366–428.
Publication types
MeSH terms
Substances
Associated data
- Actions
- Actions
- Actions
Grants and funding
LinkOut - more resources
Full Text Sources
Other Literature Sources
Molecular Biology Databases
Miscellaneous