SIRT5 Regulates both Cytosolic and Mitochondrial Protein Malonylation with Glycolysis as a Major Target
- PMID: 26073543
- PMCID: PMC4571487
- DOI: 10.1016/j.molcel.2015.05.022
SIRT5 Regulates both Cytosolic and Mitochondrial Protein Malonylation with Glycolysis as a Major Target
Abstract
Protein acylation links energetic substrate flux with cellular adaptive responses. SIRT5 is a NAD(+)-dependent lysine deacylase and removes both succinyl and malonyl groups. Using affinity enrichment and label free quantitative proteomics, we characterized the SIRT5-regulated lysine malonylome in wild-type (WT) and Sirt5(-/-) mice. 1,137 malonyllysine sites were identified across 430 proteins, with 183 sites (from 120 proteins) significantly increased in Sirt5(-/-) animals. Pathway analysis identified glycolysis as the top SIRT5-regulated pathway. Importantly, glycolytic flux was diminished in primary hepatocytes from Sirt5(-/-) compared to WT mice. Substitution of malonylated lysine residue 184 in glyceraldehyde 3-phosphate dehydrogenase with glutamic acid, a malonyllysine mimic, suppressed its enzymatic activity. Comparison with our previous reports on acylation reveals that malonylation targets a different set of proteins than acetylation and succinylation. These data demonstrate that SIRT5 is a global regulator of lysine malonylation and provide a mechanism for regulation of energetic flux through glycolysis.
Copyright © 2015 Elsevier Inc. All rights reserved.
Figures
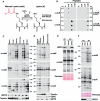
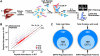
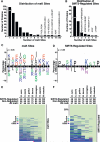
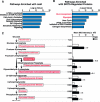
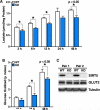
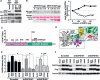
Similar articles
-
SIRT5 deacylates metabolism-related proteins and attenuates hepatic steatosis in ob/ob mice.EBioMedicine. 2018 Oct;36:347-357. doi: 10.1016/j.ebiom.2018.09.037. Epub 2018 Sep 29. EBioMedicine. 2018. PMID: 30279144 Free PMC article.
-
Protein hypoacylation induced by Sirt5 overexpression has minimal metabolic effect in mice.Biochem Biophys Res Commun. 2018 Sep 10;503(3):1349-1355. doi: 10.1016/j.bbrc.2018.07.047. Epub 2018 Jul 13. Biochem Biophys Res Commun. 2018. PMID: 30017194
-
Functions of the sirtuin deacylase SIRT5 in normal physiology and pathobiology.Crit Rev Biochem Mol Biol. 2018 Jun;53(3):311-334. doi: 10.1080/10409238.2018.1458071. Epub 2018 Apr 11. Crit Rev Biochem Mol Biol. 2018. PMID: 29637793 Free PMC article. Review.
-
The Mitochondrial Acylome Emerges: Proteomics, Regulation by Sirtuins, and Metabolic and Disease Implications.Cell Metab. 2018 Mar 6;27(3):497-512. doi: 10.1016/j.cmet.2018.01.016. Cell Metab. 2018. PMID: 29514063 Free PMC article. Review.
-
Lysine desuccinylase SIRT5 binds to cardiolipin and regulates the electron transport chain.J Biol Chem. 2017 Jun 16;292(24):10239-10249. doi: 10.1074/jbc.M117.785022. Epub 2017 Apr 30. J Biol Chem. 2017. PMID: 28458255 Free PMC article.
Cited by
-
Post-translational modifications in mitochondria: protein signaling in the powerhouse.Cell Mol Life Sci. 2016 Nov;73(21):4063-73. doi: 10.1007/s00018-016-2280-4. Epub 2016 May 27. Cell Mol Life Sci. 2016. PMID: 27233499 Free PMC article. Review.
-
The dual role of sirtuins in cancer: biological functions and implications.Front Oncol. 2024 Jun 14;14:1384928. doi: 10.3389/fonc.2024.1384928. eCollection 2024. Front Oncol. 2024. PMID: 38947884 Free PMC article. Review.
-
Sirtuins Affect Cancer Stem Cells via Epigenetic Regulation of Autophagy.Biomedicines. 2024 Feb 7;12(2):386. doi: 10.3390/biomedicines12020386. Biomedicines. 2024. PMID: 38397988 Free PMC article. Review.
-
NAMPT-Mediated NAD(+) Biosynthesis Is Essential for Vision In Mice.Cell Rep. 2016 Sep 27;17(1):69-85. doi: 10.1016/j.celrep.2016.08.073. Cell Rep. 2016. PMID: 27681422 Free PMC article.
-
Acylspermidines are conserved mitochondrial sirtuin-dependent metabolites.Nat Chem Biol. 2024 Jul;20(7):812-822. doi: 10.1038/s41589-023-01511-2. Epub 2024 Jan 2. Nat Chem Biol. 2024. PMID: 38167917
References
-
- Bao X, Zhao Q, Yang T, Fung YME, Li XD. A chemical probe for lysine malonylation. Angew. Chem. Int. Ed. Engl. 2013;52:4883–4886. - PubMed
-
- Berger SL. Histone modifications in transcriptional regulation. Curr. Opin. Genet. Dev. 2002;12:142–148. - PubMed
-
- Beysen C, Murphy EJ, McLaughlin T, Riiff T, Lamendola C, Turner HC, Awada M, Turner SM, Reaven G, Hellerstein MK. Whole-body glycolysis measured by the deuterated-glucose disposal test correlates highly with insulin resistance in vivo. Diabetes Care. 2007;30:1143–1149. - PubMed
Publication types
MeSH terms
Substances
Grants and funding
LinkOut - more resources
Full Text Sources
Other Literature Sources
Molecular Biology Databases
Research Materials