Optimization of Codon Translation Rates via tRNA Modifications Maintains Proteome Integrity
- PMID: 26052047
- PMCID: PMC4503807
- DOI: 10.1016/j.cell.2015.05.022
Optimization of Codon Translation Rates via tRNA Modifications Maintains Proteome Integrity
Abstract
Proteins begin to fold as they emerge from translating ribosomes. The kinetics of ribosome transit along a given mRNA can influence nascent chain folding, but the extent to which individual codon translation rates impact proteome integrity remains unknown. Here, we show that slower decoding of discrete codons elicits widespread protein aggregation in vivo. Using ribosome profiling, we find that loss of anticodon wobble uridine (U34) modifications in a subset of tRNAs leads to ribosome pausing at their cognate codons in S. cerevisiae and C. elegans. Cells lacking U34 modifications exhibit gene expression hallmarks of proteotoxic stress, accumulate aggregates of endogenous proteins, and are severely compromised in clearing stress-induced protein aggregates. Overexpression of hypomodified tRNAs alleviates ribosome pausing, concomitantly restoring protein homeostasis. Our findings demonstrate that modified U34 is an evolutionarily conserved accelerator of decoding and reveal an unanticipated role for tRNA modifications in maintaining proteome integrity.
Copyright © 2015 The Authors. Published by Elsevier Inc. All rights reserved.
Figures
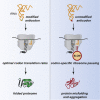
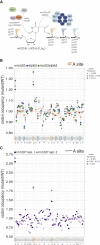
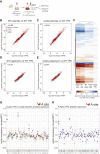
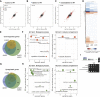
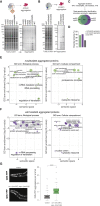
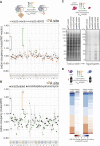
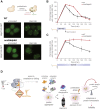
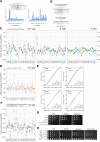
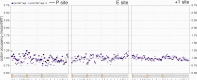
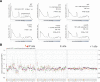
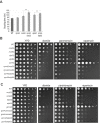
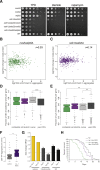
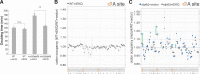
Similar articles
-
Thio-Modification of tRNA at the Wobble Position as Regulator of the Kinetics of Decoding and Translocation on the Ribosome.J Am Chem Soc. 2017 Apr 26;139(16):5857-5864. doi: 10.1021/jacs.7b00727. Epub 2017 Apr 13. J Am Chem Soc. 2017. PMID: 28368583
-
tRNA-modifying enzyme mutations induce codon-specific mistranslation and protein aggregation in yeast.RNA Biol. 2021 Apr;18(4):563-575. doi: 10.1080/15476286.2020.1819671. Epub 2020 Sep 17. RNA Biol. 2021. PMID: 32893724 Free PMC article.
-
Trm9-Catalyzed tRNA Modifications Regulate Global Protein Expression by Codon-Biased Translation.PLoS Genet. 2015 Dec 15;11(12):e1005706. doi: 10.1371/journal.pgen.1005706. eCollection 2015 Dec. PLoS Genet. 2015. PMID: 26670883 Free PMC article.
-
Bacterial wobble modifications of NNA-decoding tRNAs.IUBMB Life. 2019 Aug;71(8):1158-1166. doi: 10.1002/iub.2120. Epub 2019 Jul 8. IUBMB Life. 2019. PMID: 31283100 Free PMC article. Review.
-
Elongator, a conserved complex required for wobble uridine modifications in eukaryotes.RNA Biol. 2014;11(12):1519-28. doi: 10.4161/15476286.2014.992276. RNA Biol. 2014. PMID: 25607684 Free PMC article. Review.
Cited by
-
Roles of Elongator Dependent tRNA Modification Pathways in Neurodegeneration and Cancer.Genes (Basel). 2018 Dec 28;10(1):19. doi: 10.3390/genes10010019. Genes (Basel). 2018. PMID: 30597914 Free PMC article. Review.
-
Translational offsetting as a mode of estrogen receptor α-dependent regulation of gene expression.EMBO J. 2019 Dec 2;38(23):e101323. doi: 10.15252/embj.2018101323. Epub 2019 Sep 26. EMBO J. 2019. PMID: 31556460 Free PMC article.
-
Queuosine-modified tRNAs confer nutritional control of protein translation.EMBO J. 2018 Sep 14;37(18):e99777. doi: 10.15252/embj.201899777. Epub 2018 Aug 9. EMBO J. 2018. PMID: 30093495 Free PMC article.
-
RNA modifications modulate gene expression during development.Science. 2018 Sep 28;361(6409):1346-1349. doi: 10.1126/science.aau1646. Science. 2018. PMID: 30262497 Free PMC article. Review.
-
A tRNA modification balances carbon and nitrogen metabolism by regulating phosphate homeostasis.Elife. 2019 Jul 1;8:e44795. doi: 10.7554/eLife.44795. Elife. 2019. PMID: 31259691 Free PMC article.
References
-
- Agris P.F., Vendeix F.A.P., Graham W.D. tRNA’s wobble decoding of the genome: 40 years of modification. J. Mol. Biol. 2007;366:1–13. - PubMed
Publication types
MeSH terms
Substances
Associated data
- Actions
Grants and funding
LinkOut - more resources
Full Text Sources
Other Literature Sources
Molecular Biology Databases
Research Materials