Fluorescent-protein stabilization and high-resolution imaging of cleared, intact mouse brains
- PMID: 25993380
- PMCID: PMC4439039
- DOI: 10.1371/journal.pone.0124650
Fluorescent-protein stabilization and high-resolution imaging of cleared, intact mouse brains
Abstract
In order to observe and quantify long-range neuronal connections in intact mouse brain by light microscopy, it is first necessary to clear the brain, thus suppressing refractive-index variations. Here we describe a method that clears the brain and preserves the signal from proteinaceous fluorophores using a pH-adjusted non-aqueous index-matching medium. Successful clearing is enabled through the use of either 1-propanol or tert-butanol during dehydration whilst maintaining a basic pH. We show that high-resolution fluorescence imaging of entire, structurally intact juvenile and adult mouse brains is possible at subcellular resolution, even following many months in clearing solution. We also show that axonal long-range projections that are EGFP-labelled by modified Rabies virus can be imaged throughout the brain using a purpose-built light-sheet fluorescence microscope. To demonstrate the viability of the technique, we determined a detailed map of the monosynaptic projections onto a target cell population in the lateral entorhinal cortex. This example demonstrates that our method permits the quantification of whole-brain connectivity patterns at the subcellular level in the uncut brain.
Conflict of interest statement
Figures
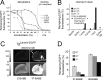
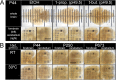
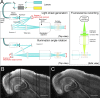
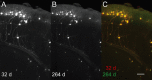
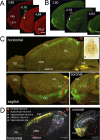
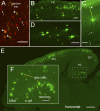
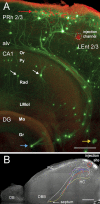
Similar articles
-
High-Speed Clearing and High-Resolution Staining for Analysis of Various Markers for Neurons and Vessels.Tissue Eng Regen Med. 2024 Oct;21(7):1037-1048. doi: 10.1007/s13770-024-00658-w. Epub 2024 Jul 2. Tissue Eng Regen Med. 2024. PMID: 38955906
-
Charting monosynaptic connectivity maps by two-color light-sheet fluorescence microscopy.Cell Rep. 2012 Nov 29;2(5):1375-86. doi: 10.1016/j.celrep.2012.10.008. Epub 2012 Nov 8. Cell Rep. 2012. PMID: 23142666
-
Light-sheet microscopy imaging of a whole cleared rat brain with Thy1-GFP transgene.Sci Rep. 2016 Jun 17;6:28209. doi: 10.1038/srep28209. Sci Rep. 2016. PMID: 27312902 Free PMC article.
-
Whole-mouse clearing and imaging at the cellular level with vDISCO.Nat Protoc. 2023 Apr;18(4):1197-1242. doi: 10.1038/s41596-022-00788-2. Epub 2023 Jan 25. Nat Protoc. 2023. PMID: 36697871 Review.
-
Whole-Brain Analysis of Cells and Circuits by Tissue Clearing and Light-Sheet Microscopy.J Neurosci. 2018 Oct 31;38(44):9330-9337. doi: 10.1523/JNEUROSCI.1677-18.2018. J Neurosci. 2018. PMID: 30381424 Free PMC article. Review.
Cited by
-
Fully Automated Evaluation of Total Glomerular Number and Capillary Tuft Size in Nephritic Kidneys Using Lightsheet Microscopy.J Am Soc Nephrol. 2017 Feb;28(2):452-459. doi: 10.1681/ASN.2016020232. Epub 2016 Aug 3. J Am Soc Nephrol. 2017. PMID: 27487796 Free PMC article.
-
High-Speed Clearing and High-Resolution Staining for Analysis of Various Markers for Neurons and Vessels.Tissue Eng Regen Med. 2024 Oct;21(7):1037-1048. doi: 10.1007/s13770-024-00658-w. Epub 2024 Jul 2. Tissue Eng Regen Med. 2024. PMID: 38955906
-
Mapping of individual sensory nerve axons from digits to spinal cord with the transparent embedding solvent system.Cell Res. 2024 Feb;34(2):124-139. doi: 10.1038/s41422-023-00867-3. Epub 2024 Jan 3. Cell Res. 2024. PMID: 38168640 Free PMC article.
-
Tissue clearing and its applications in neuroscience.Nat Rev Neurosci. 2020 Feb;21(2):61-79. doi: 10.1038/s41583-019-0250-1. Nat Rev Neurosci. 2020. PMID: 31896771 Free PMC article. Review.
-
An increase in glycoprotein concentration on extracellular virions dramatically alters vaccinia virus infectivity and pathogenesis without impacting immunogenicity.PLoS Pathog. 2021 Dec 28;17(12):e1010177. doi: 10.1371/journal.ppat.1010177. eCollection 2021 Dec. PLoS Pathog. 2021. PMID: 34962975 Free PMC article.
References
-
- Spalteholz W (1914) Über das Durchsichtigmachen von menschlichen und tierischen Präparaten und seine theoretischen Bedingungen Leipzig: S. Hierzel. 1 v. p.
-
- Dent JA, Polson AG, Klymkowsky MW (1989) A whole-mount immunocytochemical analysis of the expression of the intermediate filament protein vimentin in Xenopus. Development 105: 61–74. - PubMed
-
- Voie AH, Burns DH, Spelman FA (1993) Orthogonal-Plane Fluorescence Optical Sectioning—3-Dimensional Imaging of Macroscopic Biological Specimens. Journal of Microscopy-Oxford 170: 229–236. - PubMed
-
- Dodt HU, Leischner U, Schierloh A, Jahrling N, Mauch CP, Deininger K, et al. (2007) Ultramicroscopy: three-dimensional visualization of neuronal networks in the whole mouse brain. Nat Methods 4: 331–336. - PubMed
Publication types
MeSH terms
Substances
Grants and funding
LinkOut - more resources
Full Text Sources
Other Literature Sources