Loss of the RNA polymerase III repressor MAF1 confers obesity resistance
- PMID: 25934505
- PMCID: PMC4421982
- DOI: 10.1101/gad.258350.115
Loss of the RNA polymerase III repressor MAF1 confers obesity resistance
Abstract
MAF1 is a global repressor of RNA polymerase III transcription that regulates the expression of highly abundant noncoding RNAs in response to nutrient availability and cellular stress. Thus, MAF1 function is thought to be important for metabolic economy. Here we show that a whole-body knockout of Maf1 in mice confers resistance to diet-induced obesity and nonalcoholic fatty liver disease by reducing food intake and increasing metabolic inefficiency. Energy expenditure in Maf1(-/-) mice is increased by several mechanisms. Precursor tRNA synthesis was increased in multiple tissues without significant effects on mature tRNA levels, implying increased turnover in a futile tRNA cycle. Elevated futile cycling of hepatic lipids was also observed. Metabolite profiling of the liver and skeletal muscle revealed elevated levels of many amino acids and spermidine, which links the induction of autophagy in Maf1(-/-) mice with their extended life span. The increase in spermidine was accompanied by reduced levels of nicotinamide N-methyltransferase, which promotes polyamine synthesis, enables nicotinamide salvage to regenerate NAD(+), and is associated with obesity resistance. Consistent with this, NAD(+) levels were increased in muscle. The importance of MAF1 for metabolic economy reveals the potential for MAF1 modulators to protect against obesity and its harmful consequences.
Keywords: MAF1; RNA polymerase III; autophagy; futile cycling; metabolic efficiency; obesity; polyamines.
© 2015 Bonhoure et al.; Published by Cold Spring Harbor Laboratory Press.
Figures
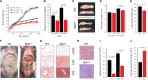
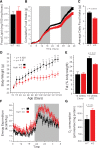
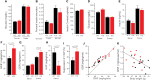
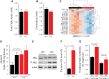
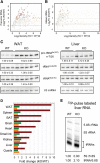
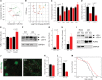
Similar articles
-
Metabolic programming a lean phenotype by deregulation of RNA polymerase III.Proc Natl Acad Sci U S A. 2018 Nov 27;115(48):12182-12187. doi: 10.1073/pnas.1815590115. Epub 2018 Nov 14. Proc Natl Acad Sci U S A. 2018. PMID: 30429315 Free PMC article.
-
MAF1 is a chronic repressor of RNA polymerase III transcription in the mouse.Sci Rep. 2020 Jul 20;10(1):11956. doi: 10.1038/s41598-020-68665-0. Sci Rep. 2020. PMID: 32686713 Free PMC article.
-
Maf1 is a novel target of PTEN and PI3K signaling that negatively regulates oncogenesis and lipid metabolism.PLoS Genet. 2014 Dec 11;10(12):e1004789. doi: 10.1371/journal.pgen.1004789. eCollection 2014 Dec. PLoS Genet. 2014. PMID: 25502566 Free PMC article.
-
Maf1 phenotypes and cell physiology.Biochim Biophys Acta Gene Regul Mech. 2018 Apr;1861(4):330-337. doi: 10.1016/j.bbagrm.2017.11.009. Epub 2017 Dec 15. Biochim Biophys Acta Gene Regul Mech. 2018. PMID: 29248739 Free PMC article. Review.
-
Emerging Roles for Maf1 beyond the Regulation of RNA Polymerase III Activity.J Mol Biol. 2015 Aug 14;427(16):2577-85. doi: 10.1016/j.jmb.2015.06.022. Epub 2015 Jul 11. J Mol Biol. 2015. PMID: 26173035 Free PMC article. Review.
Cited by
-
Roles of tRNA metabolism in aging and lifespan.Cell Death Dis. 2021 May 26;12(6):548. doi: 10.1038/s41419-021-03838-x. Cell Death Dis. 2021. PMID: 34039958 Free PMC article. Review.
-
Maf1 and Repression of RNA Polymerase III-Mediated Transcription Drive Adipocyte Differentiation.Cell Rep. 2018 Aug 14;24(7):1852-1864. doi: 10.1016/j.celrep.2018.07.046. Cell Rep. 2018. PMID: 30110641 Free PMC article.
-
Maf1 ameliorates cardiac hypertrophy by inhibiting RNA polymerase III through ERK1/2.Theranostics. 2019 Sep 25;9(24):7268-7281. doi: 10.7150/thno.33006. eCollection 2019. Theranostics. 2019. PMID: 31695767 Free PMC article.
-
Human MAF1 targets and represses active RNA polymerase III genes by preventing recruitment rather than inducing long-term transcriptional arrest.Genome Res. 2016 May;26(5):624-35. doi: 10.1101/gr.201400.115. Epub 2016 Mar 3. Genome Res. 2016. PMID: 26941251 Free PMC article.
-
DNA Methylation Profiles of Blood Cells Are Distinct between Early-Onset Obese and Control Individuals.Genomics Inform. 2017 Mar;15(1):28-37. doi: 10.5808/GI.2017.15.1.28. Epub 2017 Mar 29. Genomics Inform. 2017. PMID: 28416947 Free PMC article.
References
-
- Alexandrov A, Chernyakov I, Gu W, Hiley SL, Hughes TR, Grayhack EJ, Phizicky EM. 2006. Rapid tRNA decay can result from lack of nonessential modifications. Mol Cell 21: 87–96. - PubMed
-
- Argmann CA, Champy MF, Auwerx J. 2006. Evaluation of energy homeostasis. Curr Protoc Mol Biol 73: 29B.1.1–29B.1.17. - PubMed
Publication types
MeSH terms
Substances
Grants and funding
LinkOut - more resources
Full Text Sources
Other Literature Sources
Molecular Biology Databases
Research Materials