Ion channels in innate and adaptive immunity
- PMID: 25861976
- PMCID: PMC4822408
- DOI: 10.1146/annurev-immunol-032414-112212
Ion channels in innate and adaptive immunity
Abstract
Ion channels and transporters mediate the transport of charged ions across hydrophobic lipid membranes. In immune cells, divalent cations such as calcium, magnesium, and zinc have important roles as second messengers to regulate intracellular signaling pathways. By contrast, monovalent cations such as sodium and potassium mainly regulate the membrane potential, which indirectly controls the influx of calcium and immune cell signaling. Studies investigating human patients with mutations in ion channels and transporters, analysis of gene-targeted mice, or pharmacological experiments with ion channel inhibitors have revealed important roles of ionic signals in lymphocyte development and in innate and adaptive immune responses. We here review the mechanisms underlying the function of ion channels and transporters in lymphocytes and innate immune cells and discuss their roles in lymphocyte development, adaptive and innate immune responses, and autoimmunity, as well as recent efforts to develop pharmacological inhibitors of ion channels for immunomodulatory therapy.
Keywords: CRAC; KCa3.1; KV1.3; ORAI; STIM; TRP, T cells, B cells, macrophages, mast cells, DC, disease, therapy; calcium; chloride; ion channels; magnesium; potassium; sodium; transporters; zinc.
Figures
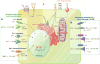
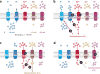
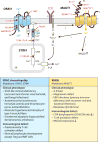
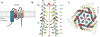
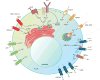
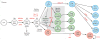
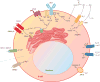
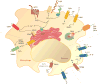
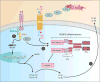
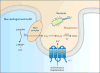
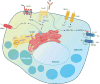
Similar articles
-
Ion channels and transporters in lymphocyte function and immunity.Nat Rev Immunol. 2012 Jun 15;12(7):532-47. doi: 10.1038/nri3233. Nat Rev Immunol. 2012. PMID: 22699833 Free PMC article. Review.
-
Immunodeficiency due to defects in store-operated calcium entry.Ann N Y Acad Sci. 2011 Nov;1238:74-90. doi: 10.1111/j.1749-6632.2011.06240.x. Ann N Y Acad Sci. 2011. PMID: 22129055 Free PMC article. Review.
-
Regulation of lymphocyte function by ORAI and STIM proteins in infection and autoimmunity.J Physiol. 2012 Sep 1;590(17):4157-67. doi: 10.1113/jphysiol.2012.233221. Epub 2012 May 21. J Physiol. 2012. PMID: 22615435 Free PMC article. Review.
-
CRAC channel regulation of innate immune cells in health and disease.Cell Calcium. 2019 Mar;78:56-65. doi: 10.1016/j.ceca.2019.01.003. Epub 2019 Jan 9. Cell Calcium. 2019. PMID: 30641250 Free PMC article. Review.
-
Beyond the CRAC: Diversification of ion signaling in B cells.Immunol Rev. 2019 Sep;291(1):104-122. doi: 10.1111/imr.12770. Immunol Rev. 2019. PMID: 31402507 Free PMC article. Review.
Cited by
-
Ca2+ Signaling but Not Store-Operated Ca2+ Entry Is Required for the Function of Macrophages and Dendritic Cells.J Immunol. 2015 Aug 1;195(3):1202-17. doi: 10.4049/jimmunol.1403013. Epub 2015 Jun 24. J Immunol. 2015. PMID: 26109647 Free PMC article.
-
Alpelisib and Immunotherapy: A Promising Combination for Recurrent and Metastatic Squamous Cell Carcinoma of the Head and Neck.Cancer Rep (Hoboken). 2024 Oct;7(10):e70023. doi: 10.1002/cnr2.70023. Cancer Rep (Hoboken). 2024. PMID: 39376013 Free PMC article.
-
Three-Dimensional Model of Sub-Plasmalemmal Ca2+ Microdomains Evoked by the Interplay Between ORAI1 and InsP3 Receptors.Front Immunol. 2021 Apr 28;12:659790. doi: 10.3389/fimmu.2021.659790. eCollection 2021. Front Immunol. 2021. PMID: 33995380 Free PMC article.
-
Impact of low sodium values on survival outcomes of patients with cancer receiving immune checkpoint inhibitors.Immunotherapy. 2024;16(12):821-828. doi: 10.1080/1750743X.2024.2370231. Epub 2024 Jul 17. Immunotherapy. 2024. PMID: 39016058
-
Mechanisms of the Immunological Effects of Volatile Anesthetics: A Review.Anesth Analg. 2016 Aug;123(2):326-35. doi: 10.1213/ANE.0000000000001403. Anesth Analg. 2016. PMID: 27308954 Free PMC article. Review.
References
Publication types
MeSH terms
Substances
Grants and funding
LinkOut - more resources
Full Text Sources
Other Literature Sources
Molecular Biology Databases