Structure of the TRPA1 ion channel suggests regulatory mechanisms
- PMID: 25855297
- PMCID: PMC4409540
- DOI: 10.1038/nature14367
Structure of the TRPA1 ion channel suggests regulatory mechanisms
Erratum in
-
Structure of the TRPA1 ion channel suggests regulatory mechanisms.Nature. 2015 Sep 24;525(7570):552. doi: 10.1038/nature14871. Epub 2015 Jul 22. Nature. 2015. PMID: 26200340 No abstract available.
Abstract
The TRPA1 ion channel (also known as the wasabi receptor) is a detector of noxious chemical agents encountered in our environment or produced endogenously during tissue injury or drug metabolism. These include a broad class of electrophiles that activate the channel through covalent protein modification. TRPA1 antagonists hold potential for treating neurogenic inflammatory conditions provoked or exacerbated by irritant exposure. Despite compelling reasons to understand TRPA1 function, structural mechanisms underlying channel regulation remain obscure. Here we use single-particle electron cryo- microscopy to determine the structure of full-length human TRPA1 to ∼4 Å resolution in the presence of pharmacophores, including a potent antagonist. Several unexpected features are revealed, including an extensive coiled-coil assembly domain stabilized by polyphosphate co-factors and a highly integrated nexus that converges on an unpredicted transient receptor potential (TRP)-like allosteric domain. These findings provide new insights into the mechanisms of TRPA1 regulation, and establish a blueprint for structure-based design of analgesic and anti-inflammatory agents.
Figures
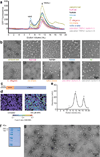
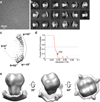
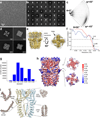
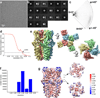
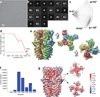
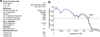
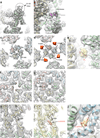
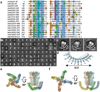
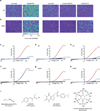
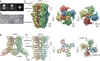
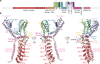
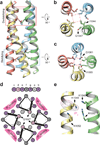
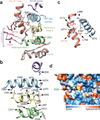
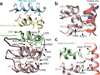
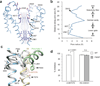
Comment in
-
Structural biology: Pain-sensing TRPA1 channel resolved.Nature. 2015 Apr 23;520(7548):439-41. doi: 10.1038/nature14383. Epub 2015 Apr 8. Nature. 2015. PMID: 25855290 No abstract available.
Similar articles
-
Structural biology: Pain-sensing TRPA1 channel resolved.Nature. 2015 Apr 23;520(7548):439-41. doi: 10.1038/nature14383. Epub 2015 Apr 8. Nature. 2015. PMID: 25855290 No abstract available.
-
How the TRPA1 receptor transmits painful stimuli: Inner workings revealed by electron cryomicroscopy.Bioessays. 2015 Nov;37(11):1184-92. doi: 10.1002/bies.201500085. Epub 2015 Sep 21. Bioessays. 2015. PMID: 26387779 Free PMC article. Review.
-
Identification of molecular determinants for a potent mammalian TRPA1 antagonist by utilizing species differences.J Mol Neurosci. 2013 Nov;51(3):754-62. doi: 10.1007/s12031-013-0060-2. Epub 2013 Jul 20. J Mol Neurosci. 2013. PMID: 23872983
-
TRPA1.Handb Exp Pharmacol. 2014;222:583-630. doi: 10.1007/978-3-642-54215-2_23. Handb Exp Pharmacol. 2014. PMID: 24756722 Review.
-
Characterization of a ligand binding site in the human transient receptor potential ankyrin 1 pore.Biophys J. 2013 Feb 19;104(4):798-806. doi: 10.1016/j.bpj.2013.01.008. Biophys J. 2013. PMID: 23442958 Free PMC article.
Cited by
-
Regulation of TRPP3 Channel Function by N-terminal Domain Palmitoylation and Phosphorylation.J Biol Chem. 2016 Dec 2;291(49):25678-25691. doi: 10.1074/jbc.M116.756544. Epub 2016 Oct 17. J Biol Chem. 2016. PMID: 27754867 Free PMC article.
-
Structural biology: Pain-sensing TRPA1 channel resolved.Nature. 2015 Apr 23;520(7548):439-41. doi: 10.1038/nature14383. Epub 2015 Apr 8. Nature. 2015. PMID: 25855290 No abstract available.
-
TRP channels in schistosomes.Int J Parasitol Drugs Drug Resist. 2016 Dec;6(3):335-342. doi: 10.1016/j.ijpddr.2016.07.002. Epub 2016 Jul 27. Int J Parasitol Drugs Drug Resist. 2016. PMID: 27496302 Free PMC article. Review.
-
A competing hydrophobic tug on L596 to the membrane core unlatches S4-S5 linker elbow from TRP helix and allows TRPV4 channel to open.Proc Natl Acad Sci U S A. 2016 Oct 18;113(42):11847-11852. doi: 10.1073/pnas.1613523113. Epub 2016 Oct 3. Proc Natl Acad Sci U S A. 2016. PMID: 27698146 Free PMC article.
-
Ion channels as therapeutic antibody targets.MAbs. 2019 Feb/Mar;11(2):265-296. doi: 10.1080/19420862.2018.1548232. Epub 2018 Dec 10. MAbs. 2019. PMID: 30526315 Free PMC article. Review.
References
-
- Julius D. TRP channels and pain. Annu Rev Cell Dev Biol. 2013;29:355–384. - PubMed
-
- Wang H, Woolf CJ. Pain TRPs. Neuron. 2005;46:9–12. - PubMed
-
- Bandell M, et al. Noxious cold ion channel TRPA1 is activated by pungent compounds and bradykinin. Neuron. 2004;41:849–857. - PubMed
-
- Bautista DM, et al. TRPA1 mediates the inflammatory actions of environmental irritants and proalgesic agents. Cell. 2006;124:1269–1282. - PubMed
Methods references
-
- Kawate T, Gouaux E. Fluorescence-detection size-exclusion chromatography for precrystallization screening of integral membrane proteins. Structure. 2006;14:673–681. - PubMed
-
- Mindell JA, Grigorieff N. Accurate determination of local defocus and specimen tilt in electron microscopy. J Struct Biol. 2003;142:334–347. - PubMed
Publication types
MeSH terms
Substances
Associated data
- Actions
Grants and funding
LinkOut - more resources
Full Text Sources
Other Literature Sources
Molecular Biology Databases