Aryl hydrocarbon receptor is necessary to protect fetal human pulmonary microvascular endothelial cells against hyperoxic injury: Mechanistic roles of antioxidant enzymes and RelB
- PMID: 25831079
- PMCID: PMC4458211
- DOI: 10.1016/j.taap.2015.03.023
Aryl hydrocarbon receptor is necessary to protect fetal human pulmonary microvascular endothelial cells against hyperoxic injury: Mechanistic roles of antioxidant enzymes and RelB
Abstract
Hyperoxia contributes to the development of bronchopulmonary dysplasia (BPD) in premature infants. Activation of the aryl hydrocarbon receptor (AhR) protects adult and newborn mice against hyperoxic lung injury by mediating increases in the expression of phase I (cytochrome P450 (CYP) 1A) and phase II (NADP(H) quinone oxidoreductase (NQO1)) antioxidant enzymes (AOE). AhR positively regulates the expression of RelB, a component of the nuclear factor-kappaB (NF-κB) protein that contributes to anti-inflammatory processes in adult animals. Whether AhR regulates the expression of AOE and RelB, and protects fetal primary human lung cells against hyperoxic injury is unknown. Therefore, we tested the hypothesis that AhR-deficient fetal human pulmonary microvascular endothelial cells (HPMEC) will have decreased RelB activation and AOE, which will in turn predispose them to increased oxidative stress, inflammation, and cell death compared to AhR-sufficient HPMEC upon exposure to hyperoxia. AhR-deficient HPMEC showed increased hyperoxia-induced reactive oxygen species (ROS) generation, cleavage of poly(ADP-ribose) polymerase (PARP), and cell death compared to AhR-sufficient HPMEC. Additionally, AhR-deficient cell culture supernatants displayed increased macrophage inflammatory protein 1α and 1β, indicating a heightened inflammatory state. Interestingly, loss of AhR was associated with a significantly attenuated CYP1A1, NQO1, superoxide dismutase 1(SOD1), and nuclear RelB protein expression. These findings support the hypothesis that decreased RelB activation and AOE in AhR-deficient cells is associated with increased hyperoxic injury compared to AhR-sufficient cells.
Keywords: Antioxidant enzymes; Aryl hydrocarbon Receptor; Fetal human pulmonary microvascular endothelial cells; Hyperoxic injury; Oxidant stress; RelB.
Copyright © 2015 Elsevier Inc. All rights reserved.
Figures
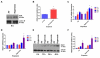
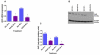
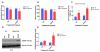
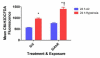
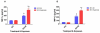
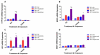
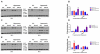
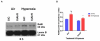
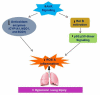
Similar articles
-
Gene Expression Profiling Identifies Cell Proliferation and Inflammation as the Predominant Pathways Regulated by Aryl Hydrocarbon Receptor in Primary Human Fetal Lung Cells Exposed to Hyperoxia.Toxicol Sci. 2016 Jul;152(1):155-68. doi: 10.1093/toxsci/kfw071. Epub 2016 Apr 21. Toxicol Sci. 2016. PMID: 27103661 Free PMC article.
-
Adrenomedullin deficiency potentiates hyperoxic injury in fetal human pulmonary microvascular endothelial cells.Biochem Biophys Res Commun. 2015 Sep 4;464(4):1048-1053. doi: 10.1016/j.bbrc.2015.07.067. Epub 2015 Jul 18. Biochem Biophys Res Commun. 2015. PMID: 26196743 Free PMC article.
-
Omeprazole induces NAD(P)H quinone oxidoreductase 1 via aryl hydrocarbon receptor-independent mechanisms: Role of the transcription factor nuclear factor erythroid 2-related factor 2.Biochem Biophys Res Commun. 2015 Nov 13;467(2):282-7. doi: 10.1016/j.bbrc.2015.09.173. Epub 2015 Oct 9. Biochem Biophys Res Commun. 2015. PMID: 26441083 Free PMC article.
-
A new cross-talk between the aryl hydrocarbon receptor and RelB, a member of the NF-kappaB family.Biochem Pharmacol. 2009 Feb 15;77(4):734-45. doi: 10.1016/j.bcp.2008.09.036. Epub 2008 Oct 8. Biochem Pharmacol. 2009. PMID: 18955032 Free PMC article. Review.
-
The Aryl Hydrocarbon Receptor (AHR): A Novel Therapeutic Target for Pulmonary Diseases?Int J Mol Sci. 2022 Jan 28;23(3):1516. doi: 10.3390/ijms23031516. Int J Mol Sci. 2022. PMID: 35163440 Free PMC article. Review.
Cited by
-
Caffeine Therapy for Apnea of Prematurity: Role of the Circadian CLOCK Gene Polymorphism.Front Pharmacol. 2022 Jan 25;12:724145. doi: 10.3389/fphar.2021.724145. eCollection 2021. Front Pharmacol. 2022. PMID: 35145399 Free PMC article.
-
Hyperglycemia-induced increasing of RELB/circ_0008590 in NF-κB pathway is repressed by miR-1243 in human retinal microvascular endothelial cells.Ann Transl Med. 2021 Nov;9(21):1624. doi: 10.21037/atm-21-5562. Ann Transl Med. 2021. PMID: 34926668 Free PMC article.
-
The Role of the Aryl Hydrocarbon Receptor (AHR) in Immune and Inflammatory Diseases.Int J Mol Sci. 2018 Dec 3;19(12):3851. doi: 10.3390/ijms19123851. Int J Mol Sci. 2018. PMID: 30513921 Free PMC article. Review.
-
Aryl Hydrocarbon Receptor: A New Player of Pathogenesis and Therapy in Cardiovascular Diseases.Biomed Res Int. 2018 Jun 10;2018:6058784. doi: 10.1155/2018/6058784. eCollection 2018. Biomed Res Int. 2018. PMID: 29984241 Free PMC article. Review.
-
Should we still use vitamin A to prevent bronchopulmonary dysplasia?J Perinatol. 2016 Aug;36(8):581-5. doi: 10.1038/jp.2016.76. Epub 2016 May 26. J Perinatol. 2016. PMID: 27228508 Review.
References
-
- Asikainen TM, White CW. Antioxidant defenses in the preterm lung: role for hypoxia-inducible factors in BPD? Toxicol Appl Pharmacol. 2005;203:177–188. - PubMed
-
- Baglole CJ, Maggirwar SB, Gasiewicz TA, Thatcher TH, Phipps RP, Sime PJ. The aryl hydrocarbon receptor attenuates tobacco smoke-induced cyclooxygenase-2 and prostaglandin production in lung fibroblasts through regulation of the NF-kappaB family member RelB. The Journal of biological chemistry. 2008;283:28944–28957. - PMC - PubMed
-
- Barazzone C, White CW. Mechanisms of cell injury and death in hyperoxia: role of cytokines and Bcl-2 family proteins. Am J Respir Cell Mol Biol. 2000;22:517–519. - PubMed
Publication types
MeSH terms
Substances
Grants and funding
- CA125123/CA/NCI NIH HHS/United States
- HD-073323/HD/NICHD NIH HHS/United States
- R01 ES009132/ES/NIEHS NIH HHS/United States
- K08 HD073323/HD/NICHD NIH HHS/United States
- AI036211/AI/NIAID NIH HHS/United States
- R01 ES019689/ES/NIEHS NIH HHS/United States
- HL-087174/HL/NHLBI NIH HHS/United States
- ES-019689/ES/NIEHS NIH HHS/United States
- HL112516/HL/NHLBI NIH HHS/United States
- R01 HL087174/HL/NHLBI NIH HHS/United States
- P30 CA125123/CA/NCI NIH HHS/United States
- R01 HL112516/HL/NHLBI NIH HHS/United States
- P30 AI036211/AI/NIAID NIH HHS/United States
- P30 ES023512/ES/NIEHS NIH HHS/United States
- ES-009132/ES/NIEHS NIH HHS/United States
- S10 RR024574/RR/NCRR NIH HHS/United States
- RR024574/RR/NCRR NIH HHS/United States
LinkOut - more resources
Full Text Sources
Other Literature Sources
Medical
Miscellaneous