Competition between RNA-binding proteins CELF1 and HuR modulates MYC translation and intestinal epithelium renewal
- PMID: 25808495
- PMCID: PMC4436827
- DOI: 10.1091/mbc.E14-11-1500
Competition between RNA-binding proteins CELF1 and HuR modulates MYC translation and intestinal epithelium renewal
Abstract
The mammalian intestinal epithelium is one of the most rapidly self-renewing tissues in the body, and its integrity is preserved through strict regulation. The RNA-binding protein (RBP) ELAV-like family member 1 (CELF1), also referred to as CUG-binding protein 1 (CUGBP1), regulates the stability and translation of target mRNAs and is implicated in many aspects of cellular physiology. We show that CELF1 competes with the RBP HuR to modulate MYC translation and regulates intestinal epithelial homeostasis. Growth inhibition of the small intestinal mucosa by fasting in mice was associated with increased CELF1/Myc mRNA association and decreased MYC expression. At the molecular level, CELF1 was found to bind the 3'-untranslated region (UTR) of Myc mRNA and repressed MYC translation without affecting total Myc mRNA levels. HuR interacted with the same Myc 3'-UTR element, and increasing the levels of HuR decreased CELF1 binding to Myc mRNA. In contrast, increasing the concentrations of CELF1 inhibited formation of the [HuR/Myc mRNA] complex. Depletion of cellular polyamines also increased CELF1 and enhanced CELF1 association with Myc mRNA, thus suppressing MYC translation. Moreover, ectopic CELF1 overexpression caused G1-phase growth arrest, whereas CELF1 silencing promoted cell proliferation. These results indicate that CELF1 represses MYC translation by decreasing Myc mRNA association with HuR and provide new insight into the molecular functions of RBPs in the regulation of intestinal mucosal growth.
© 2015 Liu, Ouyang, et al. This article is distributed by The American Society for Cell Biology under license from the author(s). Two months after publication it is available to the public under an Attribution–Noncommercial–Share Alike 3.0 Unported Creative Commons License (http://creativecommons.org/licenses/by-nc-sa/3.0).
Figures
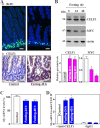
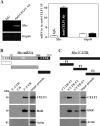
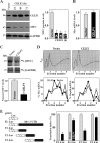
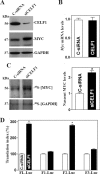
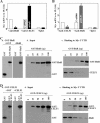
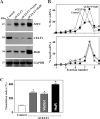
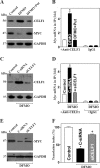
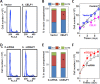
Similar articles
-
CUGBP1 and HuR regulate E-cadherin translation by altering recruitment of E-cadherin mRNA to processing bodies and modulate epithelial barrier function.Am J Physiol Cell Physiol. 2016 Jan 1;310(1):C54-65. doi: 10.1152/ajpcell.00112.2015. Epub 2015 Oct 21. Am J Physiol Cell Physiol. 2016. PMID: 26491048
-
HuR Enhances Early Restitution of the Intestinal Epithelium by Increasing Cdc42 Translation.Mol Cell Biol. 2017 Mar 17;37(7):e00574-16. doi: 10.1128/MCB.00574-16. Print 2017 Apr 1. Mol Cell Biol. 2017. PMID: 28031329 Free PMC article.
-
Polyamines regulate c-Myc translation through Chk2-dependent HuR phosphorylation.Mol Biol Cell. 2009 Dec;20(23):4885-98. doi: 10.1091/mbc.e09-07-0550. Epub 2009 Oct 7. Mol Biol Cell. 2009. PMID: 19812253 Free PMC article.
-
Properties of the Regulatory RNA-Binding Protein HuR and its Role in Controlling miRNA Repression.Adv Exp Med Biol. 2011;700:106-23. doi: 10.1007/978-1-4419-7823-3_10. Adv Exp Med Biol. 2011. PMID: 21755477 Review.
-
Properties of the regulatory RNA-binding protein HuR and its role in controlling miRNA repression.Adv Exp Med Biol. 2010;700:106-23. Adv Exp Med Biol. 2010. PMID: 21627034 Review.
Cited by
-
Small noncoding vault RNA2-1 disrupts gut epithelial barrier function via interaction with HuR.EMBO Rep. 2023 Feb 6;24(2):e54925. doi: 10.15252/embr.202254925. Epub 2022 Nov 28. EMBO Rep. 2023. PMID: 36440604 Free PMC article.
-
The Butterfly Effect of RNA Alterations on Transcriptomic Equilibrium.Cells. 2019 Dec 13;8(12):1634. doi: 10.3390/cells8121634. Cells. 2019. PMID: 31847302 Free PMC article. Review.
-
RNA-Binding Protein HuR Regulates Paneth Cell Function by Altering Membrane Localization of TLR2 via Post-transcriptional Control of CNPY3.Gastroenterology. 2019 Sep;157(3):731-743. doi: 10.1053/j.gastro.2019.05.010. Epub 2019 May 17. Gastroenterology. 2019. PMID: 31103627 Free PMC article.
-
Regulation of Intestinal Epithelial Barrier Function by Long Noncoding RNA uc.173 through Interaction with MicroRNA 29b.Mol Cell Biol. 2018 Jun 14;38(13):e00010-18. doi: 10.1128/MCB.00010-18. Print 2018 Jul 1. Mol Cell Biol. 2018. PMID: 29632078 Free PMC article.
-
Long noncoding RNA SPRY4-IT1 regulates intestinal epithelial barrier function by modulating the expression levels of tight junction proteins.Mol Biol Cell. 2016 Feb 15;27(4):617-26. doi: 10.1091/mbc.E15-10-0703. Epub 2015 Dec 17. Mol Biol Cell. 2016. PMID: 26680741 Free PMC article.
References
-
- Brook JD, McCurrach ME, Harley HG, Buckler AJ, Church D, Aburatani H, Hunter K, Stanton VP, Thirion JP, Hudson T, et al. Molecular basis of myotonic dystrophy: expansion of a trinucleotide (CTG) repeat at the 3′ end of a transcript encoding a protein kinase family member. Cell. 1992;68:799–808. - PubMed
-
- Casero RA, Jr, Marton LJ. Targeting polyamine metabolism and function in cancer and other hyperproliferative diseases. Nat Rev Drug Discov. 2007;6:373–390. - PubMed
Publication types
MeSH terms
Substances
Grants and funding
LinkOut - more resources
Full Text Sources
Research Materials
Miscellaneous