Global variability in gene expression and alternative splicing is modulated by mitochondrial content
- PMID: 25800673
- PMCID: PMC4417112
- DOI: 10.1101/gr.178426.114
Global variability in gene expression and alternative splicing is modulated by mitochondrial content
Abstract
Noise in gene expression is a main determinant of phenotypic variability. Increasing experimental evidence suggests that genome-wide cellular constraints largely contribute to the heterogeneity observed in gene products. It is still unclear, however, which global factors affect gene expression noise and to what extent. Since eukaryotic gene expression is an energy demanding process, differences in the energy budget of each cell could determine gene expression differences. Here, we quantify the contribution of mitochondrial variability (a natural source of ATP variation) to global variability in gene expression. We find that changes in mitochondrial content can account for ∼50% of the variability observed in protein levels. This is the combined result of the effect of mitochondria dosage on transcription and translation apparatus content and activities. Moreover, we find that mitochondrial levels have a large impact on alternative splicing, thus modulating both the abundance and type of mRNAs. A simple mathematical model in which mitochondrial content simultaneously affects transcription rate and splicing site choice can explain the alternative splicing data. The results of this study show that mitochondrial content (and/or probably function) influences mRNA abundance, translation, and alternative splicing, which ultimately affects cellular phenotype.
© 2015 Guantes et al.; Published by Cold Spring Harbor Laboratory Press.
Figures
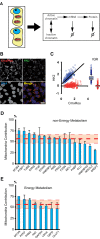
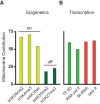
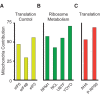
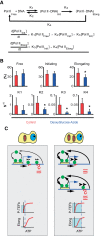
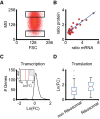
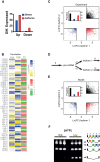
Similar articles
-
Mitochondria and the non-genetic origins of cell-to-cell variability: More is different.Bioessays. 2016 Jan;38(1):64-76. doi: 10.1002/bies.201500082. Epub 2015 Dec 12. Bioessays. 2016. PMID: 26660201 Review.
-
Regulation of the cell cycle via mitochondrial gene expression and energy metabolism in HeLa cells.Acta Biochim Biophys Sin (Shanghai). 2012 Apr;44(4):347-58. doi: 10.1093/abbs/gms006. Epub 2012 Feb 16. Acta Biochim Biophys Sin (Shanghai). 2012. PMID: 22343378
-
Mitochondrial damage modulates alternative splicing in neuronal cells: implications for neurodegeneration.J Neurochem. 2007 Jan;100(1):142-53. doi: 10.1111/j.1471-4159.2006.04204.x. Epub 2006 Oct 25. J Neurochem. 2007. PMID: 17064354
-
Nuclear and mitochondrial genome responses in HeLa cells treated with inhibitors of mitochondrial DNA expression.Acta Biochim Pol. 2006;53(3):485-95. Epub 2006 Sep 2. Acta Biochim Pol. 2006. PMID: 16951738
-
Mitochondrial topoisomerases and alternative splicing of the human TOP1mt gene.Biochimie. 2007 Apr;89(4):474-81. doi: 10.1016/j.biochi.2006.11.002. Epub 2006 Nov 27. Biochimie. 2007. PMID: 17161897 Review.
Cited by
-
HnRNPA2 is a novel histone acetyltransferase that mediates mitochondrial stress-induced nuclear gene expression.Cell Discov. 2016 Dec 6;2:16045. doi: 10.1038/celldisc.2016.45. eCollection 2016. Cell Discov. 2016. PMID: 27990297 Free PMC article.
-
Cell-to-cell variability in the propensity to transcribe explains correlated fluctuations in gene expression.Cell Syst. 2015 Nov 25;1(5):315-325. doi: 10.1016/j.cels.2015.10.011. Cell Syst. 2015. PMID: 26623441 Free PMC article.
-
Tutorial: guidelines for annotating single-cell transcriptomic maps using automated and manual methods.Nat Protoc. 2021 Jun;16(6):2749-2764. doi: 10.1038/s41596-021-00534-0. Epub 2021 May 24. Nat Protoc. 2021. PMID: 34031612 Review.
-
Systematic determination of the mitochondrial proportion in human and mice tissues for single-cell RNA-sequencing data quality control.Bioinformatics. 2021 May 17;37(7):963-967. doi: 10.1093/bioinformatics/btaa751. Bioinformatics. 2021. PMID: 32840568 Free PMC article.
-
Significance of quantitative analyses of the impact of heterogeneity in mitochondrial content and shape on cell differentiation.Open Biol. 2024 Jan;14(1):230279. doi: 10.1098/rsob.230279. Epub 2024 Jan 17. Open Biol. 2024. PMID: 38228170 Free PMC article. Review.
References
-
- Brock A, Chang H, Huang S. 2009. Non-genetic heterogeneity—a mutation-independent driving force for the somatic evolution of tumours. Nat Rev Genet 10: 336–342. - PubMed
Publication types
MeSH terms
Substances
Grants and funding
LinkOut - more resources
Full Text Sources
Other Literature Sources