Nicotinic receptor subtype-selective circuit patterns in the subthalamic nucleus
- PMID: 25740504
- PMCID: PMC4348180
- DOI: 10.1523/JNEUROSCI.3528-14.2015
Nicotinic receptor subtype-selective circuit patterns in the subthalamic nucleus
Abstract
The glutamatergic subthalamic nucleus (STN) exerts control over motor output through nuclei of the basal ganglia. High-frequency electrical stimuli in the STN effectively alleviate motor symptoms in movement disorders, and cholinergic stimulation boosts this effect. To gain knowledge about the mechanisms of cholinergic modulation in the STN, we studied cellular and circuit aspects of nicotinic acetylcholine receptors (nAChRs) in mouse STN. We discovered two largely divergent microcircuits in the STN; these are regulated in part by either α4β2 or α7 nAChRs. STN neurons containing α4β2 nAChRs (α4β2 neurons) received more glutamatergic inputs, and preferentially innervated GABAergic neurons in the substantia nigra pars reticulata. In contrast, STN neurons containing α7 nAChRs (α7 neurons) received more GABAergic inputs, and preferentially innervated dopaminergic neurons in the substantia nigra pars compacta. Interestingly, local electrical stimuli excited a majority (79%) of α4β2 neurons but exerted strong inhibition in 58% of α7 neurons, indicating an additional diversity of STN neurons: responses to electrical stimulation. Chronic exposure to nicotine selectively affects α4β2 nAChRs in STN: this treatment increased the number of α4β2 neurons, upregulated α4-containing nAChR number and sensitivity, and enhanced the basal firing rate of α4β2 neurons both ex vivo and in vivo. Thus, chronic nicotine enhances the function of the microcircuit involving α4β2 nAChRs. This indicates chronic exposure to nicotinic agonist as a potential pharmacological intervention to alter selectively the balance between these two microcircuits, and may provide a means to inhibit substantia nigra dopaminergic neurons.
Keywords: Parkinson's disease; alpha4beta2; alpha7; chronic nicotine; substantia nigra; upregulation.
Copyright © 2015 the authors 0270-6474/15/353734-13$15.00/0.
Figures
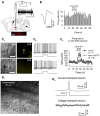
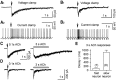
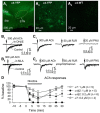
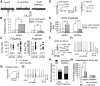
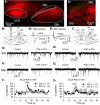
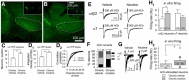
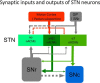
Comment in
-
Subthalamic Nucleus Cell-Specific Expression of Nicotinic Acetylcholine Receptors Uncovers Novel Basal Ganglia Microcircuits.J Neurosci. 2015 Jul 29;35(30):10645-6. doi: 10.1523/JNEUROSCI.1707-15.2015. J Neurosci. 2015. PMID: 26224850 Free PMC article. No abstract available.
Similar articles
-
Bidirectional dopamine modulation of excitatory and inhibitory synaptic inputs to subthalamic neuron subsets containing α4β2 or α7 nAChRs.Neuropharmacology. 2019 Apr;148:220-228. doi: 10.1016/j.neuropharm.2019.01.015. Epub 2019 Jan 17. Neuropharmacology. 2019. PMID: 30660626
-
Chronic nicotine selectively enhances alpha4beta2* nicotinic acetylcholine receptors in the nigrostriatal dopamine pathway.J Neurosci. 2009 Oct 7;29(40):12428-39. doi: 10.1523/JNEUROSCI.2939-09.2009. J Neurosci. 2009. PMID: 19812319 Free PMC article.
-
Prefrontal beta2 subunit-containing and alpha7 nicotinic acetylcholine receptors differentially control glutamatergic and cholinergic signaling.J Neurosci. 2010 Mar 3;30(9):3518-30. doi: 10.1523/JNEUROSCI.5712-09.2010. J Neurosci. 2010. PMID: 20203212 Free PMC article.
-
α7 nicotinic acetylcholine receptor mediated neuroprotection in Parkinson's disease.Curr Drug Targets. 2012 May;13(5):623-30. doi: 10.2174/138945012800399026. Curr Drug Targets. 2012. PMID: 22300030 Review.
-
Role for the nicotinic cholinergic system in movement disorders; therapeutic implications.Pharmacol Ther. 2014 Oct;144(1):50-9. doi: 10.1016/j.pharmthera.2014.05.004. Epub 2014 May 14. Pharmacol Ther. 2014. PMID: 24836728 Free PMC article. Review.
Cited by
-
α4 nicotinic receptors on GABAergic neurons mediate a cholinergic analgesic circuit in the substantia nigra pars reticulata.Acta Pharmacol Sin. 2024 Jun;45(6):1160-1174. doi: 10.1038/s41401-024-01234-7. Epub 2024 Mar 4. Acta Pharmacol Sin. 2024. PMID: 38438581
-
Distinct population code for movement kinematics and changes of ongoing movements in human subthalamic nucleus.Elife. 2021 Sep 14;10:e64893. doi: 10.7554/eLife.64893. Elife. 2021. PMID: 34519273 Free PMC article.
-
Parvalbumin+ and Npas1+ Pallidal Neurons Have Distinct Circuit Topology and Function.J Neurosci. 2020 Oct 7;40(41):7855-7876. doi: 10.1523/JNEUROSCI.0361-20.2020. Epub 2020 Aug 31. J Neurosci. 2020. PMID: 32868462 Free PMC article.
-
The human CHRNA7 and CHRFAM7A genes: A review of the genetics, regulation, and function.Neuropharmacology. 2015 Sep;96(Pt B):274-88. doi: 10.1016/j.neuropharm.2015.02.006. Epub 2015 Feb 19. Neuropharmacology. 2015. PMID: 25701707 Free PMC article. Review.
-
The Subthalamic Nucleus: Unravelling New Roles and Mechanisms in the Control of Action.Neuroscientist. 2019 Feb;25(1):48-64. doi: 10.1177/1073858418763594. Epub 2018 Mar 20. Neuroscientist. 2019. PMID: 29557710 Free PMC article. Review.
References
-
- Bergman H, Wichmann T, Karmon B, DeLong MR. The primate subthalamic nucleus: II. Neuronal activity in the MPTP model of parkinsonism. J Neurophysiol. 1994;72:507–520. - PubMed
Publication types
MeSH terms
Substances
Grants and funding
LinkOut - more resources
Full Text Sources
Miscellaneous