ADAR1 is required for differentiation and neural induction by regulating microRNA processing in a catalytically independent manner
- PMID: 25708366
- PMCID: PMC4387555
- DOI: 10.1038/cr.2015.24
ADAR1 is required for differentiation and neural induction by regulating microRNA processing in a catalytically independent manner
Abstract
Adenosine deaminases acting on RNA (ADARs) are involved in adenosine-to-inosine RNA editing and are implicated in development and diseases. Here we observed that ADAR1 deficiency in human embryonic stem cells (hESCs) significantly affected hESC differentiation and neural induction with widespread changes in mRNA and miRNA expression, including upregulation of self-renewal-related miRNAs, such as miR302s. Global editing analyses revealed that ADAR1 editing activity contributes little to the altered miRNA/mRNA expression in ADAR1-deficient hESCs upon neural induction. Genome-wide iCLIP studies identified that ADAR1 binds directly to pri-miRNAs to interfere with miRNA processing by acting as an RNA-binding protein. Importantly, aberrant expression of miRNAs and phenotypes observed in ADAR1-depleted hESCs upon neural differentiation could be reversed by an enzymatically inactive ADAR1 mutant, but not by the RNA-binding-null ADAR1 mutant. These findings reveal that ADAR1, but not its editing activity, is critical for hESC differentiation and neural induction by regulating miRNA biogenesis via direct RNA interaction.
Figures
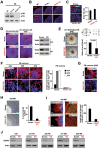
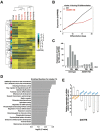
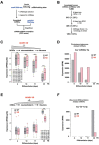
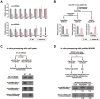
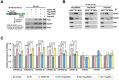
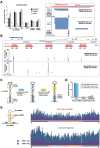
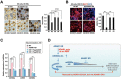
Similar articles
-
Adenosine deaminase acting on RNA-1 (ADAR1) inhibits hepatitis B virus (HBV) replication by enhancing microRNA-122 processing.J Biol Chem. 2019 Sep 20;294(38):14043-14054. doi: 10.1074/jbc.RA119.007970. Epub 2019 Jul 30. J Biol Chem. 2019. PMID: 31366735 Free PMC article.
-
Combinatory RNA-Sequencing Analyses Reveal a Dual Mode of Gene Regulation by ADAR1 in Gastric Cancer.Dig Dis Sci. 2018 Jul;63(7):1835-1850. doi: 10.1007/s10620-018-5081-9. Epub 2018 Apr 25. Dig Dis Sci. 2018. PMID: 29691780
-
Modulation of microRNA processing and expression through RNA editing by ADAR deaminases.Nat Struct Mol Biol. 2006 Jan;13(1):13-21. doi: 10.1038/nsmb1041. Epub 2005 Dec 20. Nat Struct Mol Biol. 2006. PMID: 16369484 Free PMC article.
-
A-to-I editing of coding and non-coding RNAs by ADARs.Nat Rev Mol Cell Biol. 2016 Feb;17(2):83-96. doi: 10.1038/nrm.2015.4. Epub 2015 Dec 9. Nat Rev Mol Cell Biol. 2016. PMID: 26648264 Free PMC article. Review.
-
ADAR RNA editing in innate immune response phasing, in circadian clocks and in sleep.Biochim Biophys Acta Gene Regul Mech. 2019 Mar;1862(3):356-369. doi: 10.1016/j.bbagrm.2018.10.011. Epub 2018 Oct 31. Biochim Biophys Acta Gene Regul Mech. 2019. PMID: 30391332 Review.
Cited by
-
ALU non-B-DNA conformations, flipons, binary codes and evolution.R Soc Open Sci. 2020 Jun 3;7(6):200222. doi: 10.1098/rsos.200222. eCollection 2020 Jun. R Soc Open Sci. 2020. PMID: 32742689 Free PMC article. Review.
-
Functions of the RNA Editing Enzyme ADAR1 and Their Relevance to Human Diseases.Genes (Basel). 2016 Dec 17;7(12):129. doi: 10.3390/genes7120129. Genes (Basel). 2016. PMID: 27999332 Free PMC article. Review.
-
Protein recoding by ADAR1-mediated RNA editing is not essential for normal development and homeostasis.Genome Biol. 2017 Sep 5;18(1):166. doi: 10.1186/s13059-017-1301-4. Genome Biol. 2017. PMID: 28874170 Free PMC article.
-
Improvements to the HITS-CLIP protocol eliminate widespread mispriming artifacts.BMC Genomics. 2016 May 5;17:338. doi: 10.1186/s12864-016-2675-5. BMC Genomics. 2016. PMID: 27150721 Free PMC article.
-
RNA modifications: importance in immune cell biology and related diseases.Signal Transduct Target Ther. 2022 Sep 22;7(1):334. doi: 10.1038/s41392-022-01175-9. Signal Transduct Target Ther. 2022. PMID: 36138023 Free PMC article. Review.
References
-
- Burns CM, Chu H, Rueter SM, et al. Regulation of serotonin-2C receptor G-protein coupling by RNA editing. Nature. 1997;387:303–308. - PubMed
-
- Higuchi M, Maas S, Single FN, et al. Point mutation in an AMPA receptor gene rescues lethality in mice deficient in the RNA-editing enzyme ADAR2. Nature. 2000;406:78–81. - PubMed
-
- Hoopengardner B, Bhalla T, Staber C, Reenan R. Nervous system targets of RNA editing identified by comparative genomics. Science. 2003;301:832–836. - PubMed
Publication types
MeSH terms
Substances
Associated data
- Actions
- Actions
LinkOut - more resources
Full Text Sources
Other Literature Sources
Molecular Biology Databases