LIMK1 regulates long-term memory and synaptic plasticity via the transcriptional factor CREB
- PMID: 25645926
- PMCID: PMC4372699
- DOI: 10.1128/MCB.01263-14
LIMK1 regulates long-term memory and synaptic plasticity via the transcriptional factor CREB
Abstract
Deletion of the LIMK1 gene is associated with Williams syndrome, a unique neurodevelopmental disorder characterized by severe defects in visuospatial cognition and long-term memory (LTM). However, whether LIMK1 contributes to these deficits remains elusive. Here, we show that LIMK1-knockout (LIMK1(-/-)) mice are drastically impaired in LTM but not short-term memory (STM). In addition, LIMK1(-/-) mice are selectively defective in late-phase long-term potentiation (L-LTP), a form of long-lasting synaptic plasticity specifically required for the formation of LTM. Furthermore, we show that LIMK1 interacts and regulates the activity of cyclic AMP response element-binding protein (CREB), an extensively studied transcriptional factor critical for LTM. Importantly, both L-LTP and LTM deficits in LIMK1(-/-) mice are rescued by increasing the activity of CREB. These results provide strong evidence that LIMK1 deletion is sufficient to lead to an LTM deficit and that this deficit is attributable to CREB hypofunction. Our study has identified a direct gene-phenotype link in mice and provides a potential strategy to restore LTM in patients with Williams syndrome through the enhancement of CREB activity in the adult brain.
Copyright © 2015, American Society for Microbiology. All Rights Reserved.
Figures
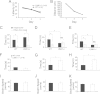
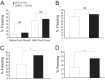
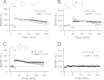
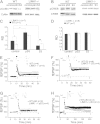
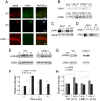
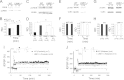
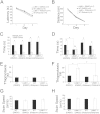
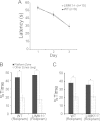
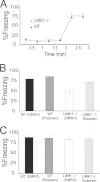
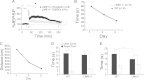
Similar articles
-
The p75 Neurotrophin Receptor Is an Essential Mediator of Impairments in Hippocampal-Dependent Associative Plasticity and Memory Induced by Sleep Deprivation.J Neurosci. 2019 Jul 10;39(28):5452-5465. doi: 10.1523/JNEUROSCI.2876-18.2019. Epub 2019 May 13. J Neurosci. 2019. PMID: 31085607 Free PMC article.
-
Upregulation of CREB-mediated transcription enhances both short- and long-term memory.J Neurosci. 2011 Jun 15;31(24):8786-802. doi: 10.1523/JNEUROSCI.3257-10.2011. J Neurosci. 2011. PMID: 21677163 Free PMC article.
-
Overexpression of LIMK1 in hippocampal excitatory neurons improves synaptic plasticity and social recognition memory in APP/PS1 mice.Mol Brain. 2021 Jul 27;14(1):121. doi: 10.1186/s13041-021-00833-3. Mol Brain. 2021. PMID: 34315506 Free PMC article.
-
LIM-Kinases in Synaptic Plasticity, Memory, and Brain Diseases.Cells. 2021 Aug 13;10(8):2079. doi: 10.3390/cells10082079. Cells. 2021. PMID: 34440848 Free PMC article. Review.
-
BDNF-induced local protein synthesis and synaptic plasticity.Neuropharmacology. 2014 Jan;76 Pt C:639-56. doi: 10.1016/j.neuropharm.2013.04.005. Epub 2013 Apr 16. Neuropharmacology. 2014. PMID: 23602987 Review.
Cited by
-
Long non-coding RNA Lnc-408 promotes invasion and metastasis of breast cancer cell by regulating LIMK1.Oncogene. 2021 Jun;40(24):4198-4213. doi: 10.1038/s41388-021-01845-y. Epub 2021 Jun 2. Oncogene. 2021. PMID: 34079084 Free PMC article.
-
Mahonia Alkaloids (MA) Ameliorate Depression Induced Gap Junction Dysfunction by miR-205/Cx43 Axis.Neurochem Res. 2022 Dec;47(12):3761-3776. doi: 10.1007/s11064-022-03761-3. Epub 2022 Oct 12. Neurochem Res. 2022. PMID: 36222958
-
Role of the hippocampal 5-HT1A receptor-mediated cAMP/PKA signalling pathway in sevoflurane-induced cognitivedysfunction in aged rats.J Int Med Res. 2018 Mar;46(3):1073-1085. doi: 10.1177/0300060517744037. Epub 2018 Jan 14. J Int Med Res. 2018. PMID: 29332488 Free PMC article.
-
The Role of ADF/Cofilin in Synaptic Physiology and Alzheimer's Disease.Front Cell Dev Biol. 2020 Nov 12;8:594998. doi: 10.3389/fcell.2020.594998. eCollection 2020. Front Cell Dev Biol. 2020. PMID: 33282872 Free PMC article. Review.
-
LncRNA Rian ameliorates sevoflurane anesthesia-induced cognitive dysfunction through regulation of miR-143-3p/LIMK1 axis.Hum Cell. 2021 May;34(3):808-818. doi: 10.1007/s13577-021-00502-6. Epub 2021 Feb 22. Hum Cell. 2021. PMID: 33616869
References
Publication types
MeSH terms
Substances
Grants and funding
LinkOut - more resources
Full Text Sources
Medical