A novel mechanism of regulating the ATPase VPS4 by its cofactor LIP5 and the endosomal sorting complex required for transport (ESCRT)-III protein CHMP5
- PMID: 25637630
- PMCID: PMC4358147
- DOI: 10.1074/jbc.M114.616730
A novel mechanism of regulating the ATPase VPS4 by its cofactor LIP5 and the endosomal sorting complex required for transport (ESCRT)-III protein CHMP5
Abstract
Disassembly of the endosomal sorting complex required for transport (ESCRT) machinery from biological membranes is a critical final step in cellular processes that require the ESCRT function. This reaction is catalyzed by VPS4, an AAA-ATPase whose activity is tightly regulated by a host of proteins, including LIP5 and the ESCRT-III proteins. Here, we present structural and functional analyses of molecular interactions between human VPS4, LIP5, and the ESCRT-III proteins. The N-terminal domain of LIP5 (LIP5NTD) is required for LIP5-mediated stimulation of VPS4, and the ESCRT-III protein CHMP5 strongly inhibits the stimulation. Both of these observations are distinct from what was previously described for homologous yeast proteins. The crystal structure of LIP5NTD in complex with the MIT (microtubule-interacting and transport)-interacting motifs of CHMP5 and a second ESCRT-III protein, CHMP1B, was determined at 1 Å resolution. It reveals an ESCRT-III binding induced moderate conformational change in LIP5NTD, which results from insertion of a conserved CHMP5 tyrosine residue (Tyr(182)) at the core of LIP5NTD structure. Mutation of Tyr(182) partially relieves the inhibition displayed by CHMP5. Together, these results suggest a novel mechanism of VPS4 regulation in metazoans, where CHMP5 functions as a negative allosteric switch to control LIP5-mediated stimulation of VPS4.
Keywords: ATPase; CHMP5; Crystal Structure; Endosomal Sorting Complexes Required for Transport (ESCRT); LIP5; MIT-MIM; Membrane Trafficking; Multivesicular Body; Protein-Protein Interaction; VPS4.
© 2015 by The American Society for Biochemistry and Molecular Biology, Inc.
Figures
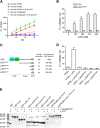
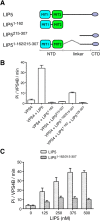
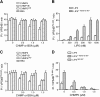
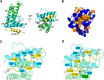
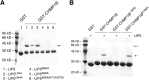
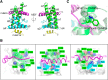
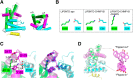
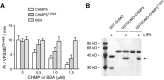
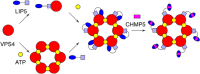
Similar articles
-
Interactions of the human LIP5 regulatory protein with endosomal sorting complexes required for transport.J Biol Chem. 2012 Dec 21;287(52):43910-26. doi: 10.1074/jbc.M112.417899. Epub 2012 Oct 26. J Biol Chem. 2012. PMID: 23105106 Free PMC article.
-
Novel interactions of ESCRT-III with LIP5 and VPS4 and their implications for ESCRT-III disassembly.Mol Biol Cell. 2008 Jun;19(6):2661-72. doi: 10.1091/mbc.e07-12-1263. Epub 2008 Apr 2. Mol Biol Cell. 2008. PMID: 18385515 Free PMC article.
-
Distinct mechanisms of recognizing endosomal sorting complex required for transport III (ESCRT-III) protein IST1 by different microtubule interacting and trafficking (MIT) domains.J Biol Chem. 2015 Mar 27;290(13):8396-408. doi: 10.1074/jbc.M114.607903. Epub 2015 Feb 5. J Biol Chem. 2015. PMID: 25657007 Free PMC article.
-
Meiotic Clade AAA ATPases: Protein Polymer Disassembly Machines.J Mol Biol. 2016 May 8;428(9 Pt B):1897-911. doi: 10.1016/j.jmb.2015.11.004. Epub 2015 Nov 10. J Mol Biol. 2016. PMID: 26555750 Free PMC article. Review.
-
The role of VPS4 in ESCRT-III polymer remodeling.Biochem Soc Trans. 2019 Feb 28;47(1):441-448. doi: 10.1042/BST20180026. Epub 2019 Feb 19. Biochem Soc Trans. 2019. PMID: 30783012 Review.
Cited by
-
ESCRT Requirements for Murine Leukemia Virus Release.Viruses. 2016 Apr 18;8(4):103. doi: 10.3390/v8040103. Viruses. 2016. PMID: 27096867 Free PMC article.
-
Mechanism of cargo sorting into small extracellular vesicles.Bioengineered. 2021 Dec;12(1):8186-8201. doi: 10.1080/21655979.2021.1977767. Bioengineered. 2021. PMID: 34661500 Free PMC article.
-
Identification and Characterization of BmVta1, a Bombyx mori (Lepidoptera: Bombycidae) Homologue for Vta1 That is Up-Regulated in Development.J Insect Sci. 2017 May 1;17(3):79. doi: 10.1093/jisesa/iex055. J Insect Sci. 2017. PMID: 28973578 Free PMC article.
-
NMR studies on the interactions between yeast Vta1 and Did2 during the multivesicular bodies sorting pathway.Sci Rep. 2016 Dec 7;6:38710. doi: 10.1038/srep38710. Sci Rep. 2016. PMID: 27924850 Free PMC article.
-
Structural basis of protein translocation by the Vps4-Vta1 AAA ATPase.Elife. 2017 Apr 5;6:e24487. doi: 10.7554/eLife.24487. Elife. 2017. PMID: 28379137 Free PMC article.
References
-
- Henne W. M., Buchkovich N. J., Emr S. D. (2011) The ESCRT pathway. Dev. Cell 21, 77–91 - PubMed
-
- Hanson P. I., Cashikar A. (2012) Multivesicular body morphogenesis. Annu. Rev. Cell Dev. Biol. 28, 337–362 - PubMed
-
- Katzmann D. J., Babst M., Emr S. D. (2001) Ubiquitin-dependent sorting into the multivesicular body pathway requires the function of a conserved endosomal protein sorting complex, ESCRT-I. Cell 106, 145–155 - PubMed
Publication types
MeSH terms
Substances
Associated data
- Actions
- Actions
- Actions
Grants and funding
LinkOut - more resources
Full Text Sources
Molecular Biology Databases
Miscellaneous