Spartan deficiency causes genomic instability and progeroid phenotypes
- PMID: 25501849
- PMCID: PMC4269170
- DOI: 10.1038/ncomms6744
Spartan deficiency causes genomic instability and progeroid phenotypes
Abstract
Spartan (also known as DVC1 and C1orf124) is a PCNA-interacting protein implicated in translesion synthesis, a DNA damage tolerance process that allows the DNA replication machinery to replicate past nucleotide lesions. However, the physiological relevance of Spartan has not been established. Here we report that Spartan insufficiency in mice causes chromosomal instability, cellular senescence and early onset of age-related phenotypes. Whereas complete loss of Spartan causes early embryonic lethality, hypomorphic mice with low amounts of Spartan are viable. These mice are growth retarded and develop cataracts, lordokyphosis and cachexia at a young age. Cre-mediated depletion of Spartan from conditional knockout mouse embryonic fibroblasts results in impaired lesion bypass, incomplete DNA replication, formation of micronuclei and chromatin bridges and eventually cell death. These data demonstrate that Spartan plays a key role in maintaining structural and numerical chromosome integrity and suggest a link between Spartan insufficiency and progeria.
Figures
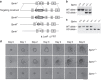
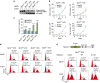
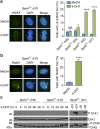
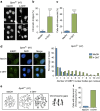
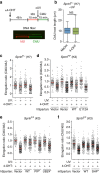
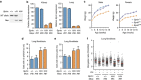
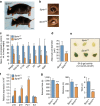
Similar articles
-
Spartan deficiency causes accumulation of Topoisomerase 1 cleavage complexes and tumorigenesis.Nucleic Acids Res. 2017 May 5;45(8):4564-4576. doi: 10.1093/nar/gkx107. Nucleic Acids Res. 2017. PMID: 28199696 Free PMC article.
-
Defective ATM-Kap-1-mediated chromatin remodeling impairs DNA repair and accelerates senescence in progeria mouse model.Aging Cell. 2013 Apr;12(2):316-8. doi: 10.1111/acel.12035. Epub 2012 Dec 21. Aging Cell. 2013. PMID: 23173799
-
Spartan/C1orf124 is important to prevent UV-induced mutagenesis.Cell Cycle. 2012 Sep 15;11(18):3395-402. doi: 10.4161/cc.21694. Epub 2012 Aug 16. Cell Cycle. 2012. PMID: 22894931 Free PMC article.
-
Unraveling the mysteries of aging through a Hutchinson-Gilford progeria syndrome model.Rejuvenation Res. 2011 Apr;14(2):133-41. doi: 10.1089/rej.2010.1088. Epub 2011 Jan 5. Rejuvenation Res. 2011. PMID: 21208065 Review.
-
From the rarest to the most common: insights from progeroid syndromes into skin cancer and aging.J Invest Dermatol. 2009 Oct;129(10):2340-50. doi: 10.1038/jid.2009.103. Epub 2009 Apr 23. J Invest Dermatol. 2009. PMID: 19387478 Review.
Cited by
-
The CMG Helicase Bypasses DNA-Protein Cross-Links to Facilitate Their Repair.Cell. 2019 Jan 10;176(1-2):167-181.e21. doi: 10.1016/j.cell.2018.10.053. Epub 2018 Dec 27. Cell. 2019. PMID: 30595447 Free PMC article.
-
A model of DNA damage response activation at stalled replication forks by SPRTN.Nat Commun. 2019 Dec 12;10(1):5671. doi: 10.1038/s41467-019-13610-7. Nat Commun. 2019. PMID: 31831745 Free PMC article.
-
DNA-protein cross-link repair: what do we know now?Cell Biosci. 2020 Jan 7;10:3. doi: 10.1186/s13578-019-0366-z. eCollection 2020. Cell Biosci. 2020. PMID: 31921408 Free PMC article. Review.
-
Genomic instability and DNA replication defects in progeroid syndromes.Nucleus. 2018 Dec 31;9(1):368-379. doi: 10.1080/19491034.2018.1476793. Epub 2018 Jun 23. Nucleus. 2018. PMID: 29936894 Free PMC article. Review.
-
Transcription-coupled repair of DNA-protein cross-links depends on CSA and CSB.Nat Cell Biol. 2024 May;26(5):797-810. doi: 10.1038/s41556-024-01391-1. Epub 2024 Apr 10. Nat Cell Biol. 2024. PMID: 38600235 Free PMC article.
References
Publication types
MeSH terms
Substances
Grants and funding
LinkOut - more resources
Full Text Sources
Other Literature Sources
Medical
Molecular Biology Databases
Miscellaneous