All brains are made of this: a fundamental building block of brain matter with matching neuronal and glial masses
- PMID: 25429260
- PMCID: PMC4228857
- DOI: 10.3389/fnana.2014.00127
All brains are made of this: a fundamental building block of brain matter with matching neuronal and glial masses
Abstract
How does the size of the glial and neuronal cells that compose brain tissue vary across brain structures and species? Our previous studies indicate that average neuronal size is highly variable, while average glial cell size is more constant. Measuring whole cell sizes in vivo, however, is a daunting task. Here we use chi-square minimization of the relationship between measured neuronal and glial cell densities in the cerebral cortex, cerebellum, and rest of brain in 27 mammalian species to model neuronal and glial cell mass, as well as the neuronal mass fraction of the tissue (the fraction of tissue mass composed by neurons). Our model shows that while average neuronal cell mass varies by over 500-fold across brain structures and species, average glial cell mass varies only 1.4-fold. Neuronal mass fraction varies typically between 0.6 and 0.8 in all structures. Remarkably, we show that two fundamental, universal relationships apply across all brain structures and species: (1) the glia/neuron ratio varies with the total neuronal mass in the tissue (which in turn depends on variations in average neuronal cell mass), and (2) the neuronal mass per glial cell, and with it the neuronal mass fraction and neuron/glia mass ratio, varies with average glial cell mass in the tissue. We propose that there is a fundamental building block of brain tissue: the glial mass that accompanies a unit of neuronal mass. We argue that the scaling of this glial mass is a consequence of a universal mechanism whereby numbers of glial cells are added to the neuronal parenchyma during development, irrespective of whether the neurons composing it are large or small, but depending on the average mass of the glial cells being added. We also show how evolutionary variations in neuronal cell mass, glial cell mass and number of neurons suffice to determine the most basic characteristics of brain structures, such as mass, glia/neuron ratio, neuron/glia mass ratio, and cell densities.
Keywords: allometry; brain size; cell size; glia/neuron ratio; number of glial cells; number of neurons.
Figures
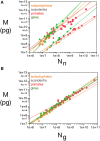
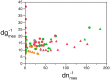
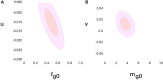
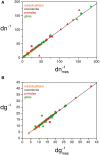
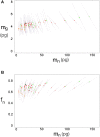
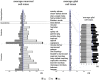
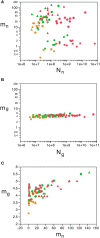
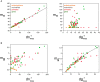
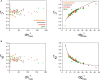
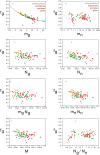
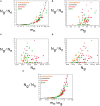
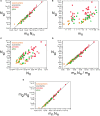
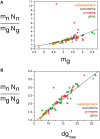
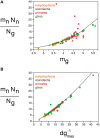
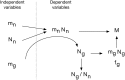
Similar articles
-
Not all brains are made the same: new views on brain scaling in evolution.Brain Behav Evol. 2011;78(1):22-36. doi: 10.1159/000327318. Epub 2011 Jun 17. Brain Behav Evol. 2011. PMID: 21691045 Review.
-
The glia/neuron ratio: how it varies uniformly across brain structures and species and what that means for brain physiology and evolution.Glia. 2014 Sep;62(9):1377-91. doi: 10.1002/glia.22683. Epub 2014 May 7. Glia. 2014. PMID: 24807023 Review.
-
Cellular scaling rules for rodent brains.Proc Natl Acad Sci U S A. 2006 Aug 8;103(32):12138-43. doi: 10.1073/pnas.0604911103. Epub 2006 Jul 31. Proc Natl Acad Sci U S A. 2006. PMID: 16880386 Free PMC article.
-
Brain scaling in mammalian evolution as a consequence of concerted and mosaic changes in numbers of neurons and average neuronal cell size.Front Neuroanat. 2014 Aug 11;8:77. doi: 10.3389/fnana.2014.00077. eCollection 2014. Front Neuroanat. 2014. PMID: 25157220 Free PMC article. Review.
-
Cellular Scaling Rules for the Brains of Marsupials: Not as "Primitive" as Expected.Brain Behav Evol. 2017;89(1):48-63. doi: 10.1159/000452856. Epub 2017 Jan 27. Brain Behav Evol. 2017. PMID: 28125804
Cited by
-
When larger brains do not have more neurons: increased numbers of cells are compensated by decreased average cell size across mouse individuals.Front Neuroanat. 2015 Jun 1;9:64. doi: 10.3389/fnana.2015.00064. eCollection 2015. Front Neuroanat. 2015. PMID: 26082686 Free PMC article.
-
Neuro-evolutionary evidence for a universal fractal primate brain shape.Elife. 2024 Sep 30;12:RP92080. doi: 10.7554/eLife.92080. Elife. 2024. PMID: 39347569 Free PMC article.
-
Neuronal and non-neuronal scaling across brain regions within an intercross of domestic and wild chickens.Front Neuroanat. 2022 Nov 25;16:1048261. doi: 10.3389/fnana.2022.1048261. eCollection 2022. Front Neuroanat. 2022. PMID: 36506870 Free PMC article.
-
Similar Microglial Cell Densities across Brain Structures and Mammalian Species: Implications for Brain Tissue Function.J Neurosci. 2020 Jun 10;40(24):4622-4643. doi: 10.1523/JNEUROSCI.2339-19.2020. Epub 2020 Apr 6. J Neurosci. 2020. PMID: 32253358 Free PMC article.
-
Detecting Microglial Density With Quantitative Multi-Compartment Diffusion MRI.Front Neurosci. 2019 Feb 19;13:81. doi: 10.3389/fnins.2019.00081. eCollection 2019. Front Neurosci. 2019. PMID: 30837826 Free PMC article.
References
-
- Braitenberg V., Schüz A. (1998). Cortex: Statistic and Geometry of Neuronal Connectivity, 2nd Edn. New York, NY: Springer-Verlag.
LinkOut - more resources
Full Text Sources
Other Literature Sources