Actin polymerization as a key innate immune effector mechanism to control Salmonella infection
- PMID: 25422455
- PMCID: PMC4267384
- DOI: 10.1073/pnas.1419925111
Actin polymerization as a key innate immune effector mechanism to control Salmonella infection
Abstract
Salmonellosis is one of the leading causes of food poisoning worldwide. Controlling bacterial burden is essential to surviving infection. Nucleotide-binding oligomerization domain-like receptors (NLRs), such as NLRC4, induce inflammasome effector functions and play a crucial role in controlling Salmonella infection. Inflammasome-dependent production of IL-1β recruits additional immune cells to the site of infection, whereas inflammasome-mediated pyroptosis of macrophages releases bacteria for uptake by neutrophils. Neither of these functions is known to directly kill intracellular salmonellae within macrophages. The mechanism, therefore, governing how inflammasomes mediate intracellular bacterial-killing and clearance in host macrophages remains unknown. Here, we show that actin polymerization is required for NLRC4-dependent regulation of intracellular bacterial burden, inflammasome assembly, pyroptosis, and IL-1β production. NLRC4-induced changes in actin polymerization are physically manifested as increased cellular stiffness, and leads to reduced bacterial uptake, production of antimicrobial molecules, and arrested cellular migration. These processes act in concert to limit bacterial replication in the cell and dissemination in tissues. We show, therefore, a functional link between innate immunity and actin turnover in macrophages that underpins a key host defense mechanism for the control of salmonellosis.
Keywords: ASC; ROS; caspase-1; cytoskeleton; innate immunity.
Conflict of interest statement
The authors declare no conflict of interest.
Figures
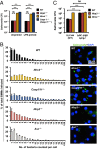
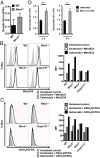
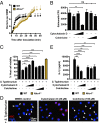
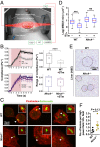
Comment in
-
Inflammasome: Stiffening up defences against Salmonella.Nat Rev Immunol. 2015 Jan;15(1):4. doi: 10.1038/nri3798. Nat Rev Immunol. 2015. PMID: 25534618 No abstract available.
Similar articles
-
The neutrophil NLRC4 inflammasome selectively promotes IL-1β maturation without pyroptosis during acute Salmonella challenge.Cell Rep. 2014 Jul 24;8(2):570-82. doi: 10.1016/j.celrep.2014.06.028. Epub 2014 Jul 17. Cell Rep. 2014. PMID: 25043180
-
Caspase-1-induced pyroptosis is an innate immune effector mechanism against intracellular bacteria.Nat Immunol. 2010 Dec;11(12):1136-42. doi: 10.1038/ni.1960. Epub 2010 Nov 7. Nat Immunol. 2010. PMID: 21057511 Free PMC article.
-
Inflammasome activation causes dual recruitment of NLRC4 and NLRP3 to the same macromolecular complex.Proc Natl Acad Sci U S A. 2014 May 20;111(20):7403-8. doi: 10.1073/pnas.1402911111. Epub 2014 May 6. Proc Natl Acad Sci U S A. 2014. PMID: 24803432 Free PMC article.
-
Advances in Understanding Activation and Function of the NLRC4 Inflammasome.Int J Mol Sci. 2021 Jan 21;22(3):1048. doi: 10.3390/ijms22031048. Int J Mol Sci. 2021. PMID: 33494299 Free PMC article. Review.
-
Salmonella and the Inflammasome: Battle for Intracellular Dominance.Curr Top Microbiol Immunol. 2016;397:43-67. doi: 10.1007/978-3-319-41171-2_3. Curr Top Microbiol Immunol. 2016. PMID: 27460804 Review.
Cited by
-
Compliant Substrates Enhance Macrophage Cytokine Release and NLRP3 Inflammasome Formation During Their Pro-Inflammatory Response.Front Cell Dev Biol. 2021 Mar 29;9:639815. doi: 10.3389/fcell.2021.639815. eCollection 2021. Front Cell Dev Biol. 2021. PMID: 33855019 Free PMC article.
-
Proteomic analysis of crocodile white blood cells reveals insights into the mechanism of the innate immune system.Heliyon. 2024 Jan 17;10(2):e24583. doi: 10.1016/j.heliyon.2024.e24583. eCollection 2024 Jan 30. Heliyon. 2024. PMID: 38312682 Free PMC article.
-
Downregulation of calponin 2 contributes to the quiescence of lung macrophages.Am J Physiol Cell Physiol. 2019 Oct 1;317(4):C749-C761. doi: 10.1152/ajpcell.00036.2019. Epub 2019 Jul 31. Am J Physiol Cell Physiol. 2019. PMID: 31365293 Free PMC article.
-
SipA Activation of Caspase-3 Is a Decisive Mediator of Host Cell Survival at Early Stages of Salmonella enterica Serovar Typhimurium Infection.Infect Immun. 2017 Aug 18;85(9):e00393-17. doi: 10.1128/IAI.00393-17. Print 2017 Sep. Infect Immun. 2017. PMID: 28630067 Free PMC article.
-
Bacterial detection by NAIP/NLRC4 elicits prompt contractions of intestinal epithelial cell layers.Proc Natl Acad Sci U S A. 2021 Apr 20;118(16):e2013963118. doi: 10.1073/pnas.2013963118. Proc Natl Acad Sci U S A. 2021. PMID: 33846244 Free PMC article.
References
-
- Aderem A, Underhill DM. Mechanisms of phagocytosis in macrophages. Annu Rev Immunol. 1999;17:593–623. - PubMed
-
- Mastroeni P, Grant A, Restif O, Maskell D. A dynamic view of the spread and intracellular distribution of Salmonella enterica. Nat Rev Microbiol. 2009;7(1):73–80. - PubMed
-
- Haraga A, Ohlson MB, Miller SI. Salmonellae interplay with host cells. Nat Rev Microbiol. 2008;6(1):53–66. - PubMed
Publication types
MeSH terms
Substances
Grants and funding
- 282060/ERC_/European Research Council/International
- BB/H003916/1/BB_/Biotechnology and Biological Sciences Research Council/United Kingdom
- BB/K006436/1/BB_/Biotechnology and Biological Sciences Research Council/United Kingdom
- BB/H021930/1/BB_/Biotechnology and Biological Sciences Research Council/United Kingdom
LinkOut - more resources
Full Text Sources
Other Literature Sources
Medical
Molecular Biology Databases
Miscellaneous