The talin head domain reinforces integrin-mediated adhesion by promoting adhesion complex stability and clustering
- PMID: 25393120
- PMCID: PMC4230843
- DOI: 10.1371/journal.pgen.1004756
The talin head domain reinforces integrin-mediated adhesion by promoting adhesion complex stability and clustering
Abstract
Talin serves an essential function during integrin-mediated adhesion in linking integrins to actin via the intracellular adhesion complex. In addition, the N-terminal head domain of talin regulates the affinity of integrins for their ECM-ligands, a process known as inside-out activation. We previously showed that in Drosophila, mutating the integrin binding site in the talin head domain resulted in weakened adhesion to the ECM. Intriguingly, subsequent studies showed that canonical inside-out activation of integrin might not take place in flies. Consistent with this, a mutation in talin that specifically blocks its ability to activate mammalian integrins does not significantly impinge on talin function during fly development. Here, we describe results suggesting that the talin head domain reinforces and stabilizes the integrin adhesion complex by promoting integrin clustering distinct from its ability to support inside-out activation. Specifically, we show that an allele of talin containing a mutation that disrupts intramolecular interactions within the talin head attenuates the assembly and reinforcement of the integrin adhesion complex. Importantly, we provide evidence that this mutation blocks integrin clustering in vivo. We propose that the talin head domain is essential for regulating integrin avidity in Drosophila and that this is crucial for integrin-mediated adhesion during animal development.
Conflict of interest statement
The authors have declared that no competing interests exist.
Figures
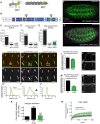
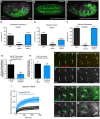
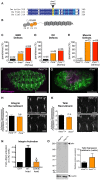
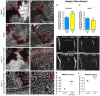
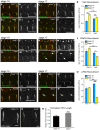
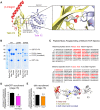
Similar articles
-
Direct binding of Talin to Rap1 is required for cell-ECM adhesion in Drosophila.J Cell Sci. 2018 Dec 18;131(24):jcs225144. doi: 10.1242/jcs.225144. J Cell Sci. 2018. PMID: 30446511
-
In vivo functional analysis reveals specific roles for the integrin-binding sites of talin.J Cell Sci. 2011 Jun 1;124(Pt 11):1844-56. doi: 10.1242/jcs.083337. Epub 2011 May 10. J Cell Sci. 2011. PMID: 21558413
-
An interaction between integrin and the talin FERM domain mediates integrin activation but not linkage to the cytoskeleton.Nat Cell Biol. 2006 Jun;8(6):601-6. doi: 10.1038/ncb1411. Epub 2006 Apr 30. Nat Cell Biol. 2006. PMID: 16648844
-
Talin - the master of integrin adhesions.J Cell Sci. 2017 Aug 1;130(15):2435-2446. doi: 10.1242/jcs.190991. Epub 2017 Jul 12. J Cell Sci. 2017. PMID: 28701514 Review.
-
Mechanisms of talin-dependent integrin signaling and crosstalk.Biochim Biophys Acta. 2014 Feb;1838(2):579-88. doi: 10.1016/j.bbamem.2013.07.017. Epub 2013 Jul 24. Biochim Biophys Acta. 2014. PMID: 23891718 Free PMC article. Review.
Cited by
-
Talin1 is required for cardiac Z-disk stabilization and endothelial integrity in zebrafish.FASEB J. 2015 Dec;29(12):4989-5005. doi: 10.1096/fj.15-273409. Epub 2015 Aug 26. FASEB J. 2015. PMID: 26310270 Free PMC article.
-
Cancer associated talin point mutations disorganise cell adhesion and migration.Sci Rep. 2021 Jan 11;11(1):347. doi: 10.1038/s41598-020-77911-4. Sci Rep. 2021. PMID: 33431906 Free PMC article.
-
Regulation of integrin-mediated adhesions.Curr Opin Cell Biol. 2015 Oct;36:41-7. doi: 10.1016/j.ceb.2015.06.009. Epub 2015 Jul 17. Curr Opin Cell Biol. 2015. PMID: 26189062 Free PMC article. Review.
-
The role of integrins in inflammation and angiogenesis.Pediatr Res. 2021 May;89(7):1619-1626. doi: 10.1038/s41390-020-01177-9. Epub 2020 Oct 7. Pediatr Res. 2021. PMID: 33027803 Free PMC article. Review.
-
Talin variant P229S compromises integrin activation and associates with multifaceted clinical symptoms.Hum Mol Genet. 2022 Dec 16;31(24):4159-4172. doi: 10.1093/hmg/ddac163. Hum Mol Genet. 2022. PMID: 35861643 Free PMC article.
References
-
- Bokel C, Brown NH (2002) Integrins in development: moving on, responding to, and sticking to the extracellular matrix. Dev Cell 3: 311–321. - PubMed
Publication types
MeSH terms
Substances
Grants and funding
LinkOut - more resources
Full Text Sources
Other Literature Sources
Molecular Biology Databases