Chemical conversion of human fibroblasts into functional Schwann cells
- PMID: 25358782
- PMCID: PMC4223700
- DOI: 10.1016/j.stemcr.2014.07.014
Chemical conversion of human fibroblasts into functional Schwann cells
Abstract
Direct transdifferentiation of somatic cells is a promising approach to obtain patient-specific cells for numerous applications. However, conversion across germ-layer borders often requires ectopic gene expression with unpredictable side effects. Here, we present a gene-free approach that allows efficient conversion of human fibroblasts via a transient progenitor stage into Schwann cells, the major glial cell type of peripheral nerves. Using a multikinase inhibitor, we transdifferentiated fibroblasts into transient neural precursors that were subsequently further differentiated into Schwann cells. The resulting induced Schwann cells (iSCs) expressed numerous Schwann cell-specific proteins and displayed neurosupportive and myelination capacity in vitro. Thus, we established a strategy to obtain mature Schwann cells from human postnatal fibroblasts under chemically defined conditions without the introduction of ectopic genes.
Copyright © 2014 The Authors. Published by Elsevier Inc. All rights reserved.
Figures
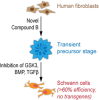
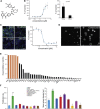
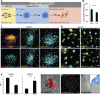
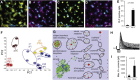
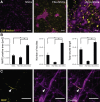
Similar articles
-
Chemical Conversion of Human Fibroblasts into Functional Schwann Cells.Methods Mol Biol. 2018;1739:127-136. doi: 10.1007/978-1-4939-7649-2_8. Methods Mol Biol. 2018. PMID: 29546704
-
Direct conversion of adult human skin fibroblasts into functional Schwann cells that achieve robust recovery of the severed peripheral nerve in rats.Glia. 2019 May;67(5):950-966. doi: 10.1002/glia.23582. Epub 2019 Jan 13. Glia. 2019. PMID: 30637802
-
Proteomic analysis of mesenchymal to Schwann cell transdifferentiation.J Proteomics. 2017 Aug 8;165:93-101. doi: 10.1016/j.jprot.2017.06.011. Epub 2017 Jun 17. J Proteomics. 2017. PMID: 28629798
-
[Phenotypic plasticity of neural crest-derived melanocytes and Schwann cells].Biol Aujourdhui. 2011;205(1):53-61. doi: 10.1051/jbio/2011008. Epub 2011 Apr 19. Biol Aujourdhui. 2011. PMID: 21501576 Review. French.
-
Signals that determine Schwann cell identity.J Anat. 2002 Apr;200(4):367-76. doi: 10.1046/j.1469-7580.2002.00046.x. J Anat. 2002. PMID: 12090403 Free PMC article. Review.
Cited by
-
Biomimetic Strategies for Peripheral Nerve Injury Repair: An Exploration of Microarchitecture and Cellularization.Biomed Mater Devices. 2023 Mar;1(1):21-37. doi: 10.1007/s44174-022-00039-8. Epub 2022 Oct 3. Biomed Mater Devices. 2023. PMID: 38343513 Free PMC article.
-
Clinical Trials in a Dish: The Potential of Pluripotent Stem Cells to Develop Therapies for Neurodegenerative Diseases.Annu Rev Pharmacol Toxicol. 2016;56:489-510. doi: 10.1146/annurev-pharmtox-010715-103548. Epub 2015 Oct 28. Annu Rev Pharmacol Toxicol. 2016. PMID: 26514199 Free PMC article. Review.
-
Egr2 overexpression in Schwann cells increases myelination frequency in vitro.Heliyon. 2018 Nov 29;4(11):e00982. doi: 10.1016/j.heliyon.2018.e00982. eCollection 2018 Nov. Heliyon. 2018. PMID: 30761371 Free PMC article.
-
Derivation of Adult Human Fibroblasts and their Direct Conversion into Expandable Neural Progenitor Cells.J Vis Exp. 2015 Jul 29;(101):e52831. doi: 10.3791/52831. J Vis Exp. 2015. PMID: 26275015 Free PMC article.
-
Development and In Vitro Differentiation of Schwann Cells.Cells. 2022 Nov 24;11(23):3753. doi: 10.3390/cells11233753. Cells. 2022. PMID: 36497014 Free PMC article. Review.
References
-
- Baker M.D. Electrophysiology of mammalian Schwann cells. Prog. Biophys. Mol. Biol. 2002;78:83–103. - PubMed
-
- Bhatheja K., Field J. Schwann cells: origins and role in axonal maintenance and regeneration. Int. J. Biochem. Cell Biol. 2006;38:1995–1999. - PubMed
-
- Bronner-Fraser M. An antibody to a receptor for fibronectin and laminin perturbs cranial neural crest development in vivo. Dev. Biol. 1986;117:528–536. - PubMed
-
- Casella G.T., Bunge R.P., Wood P.M. Improved method for harvesting human Schwann cells from mature peripheral nerve and expansion in vitro. Glia. 1996;17:327–338. - PubMed
Publication types
MeSH terms
Substances
Associated data
- Actions
LinkOut - more resources
Full Text Sources
Other Literature Sources
Molecular Biology Databases