Investigation of interactions at the extracellular loops of the relaxin family peptide receptor 1 (RXFP1)
- PMID: 25352603
- PMCID: PMC4263891
- DOI: 10.1074/jbc.M114.600882
Investigation of interactions at the extracellular loops of the relaxin family peptide receptor 1 (RXFP1)
Abstract
Relaxin, an emerging pharmaceutical treatment for acute heart failure, activates the relaxin family peptide receptor (RXFP1), which is a class A G-protein-coupled receptor. In addition to the classic transmembrane (TM) domain, RXFP1 possesses a large extracellular domain consisting of 10 leucine-rich repeats and an N-terminal low density lipoprotein class A (LDLa) module. Relaxin-mediated activation of RXFP1 requires multiple coordinated interactions between the ligand and various receptor domains including a high affinity interaction involving the leucine-rich repeats and a predicted lower affinity interaction involving the extracellular loops (ELs). The LDLa is essential for signal activation; therefore the ELs/TM may additionally present an interaction site to facilitate this LDLa-mediated signaling. To overcome the many challenges of investigating relaxin and the LDLa module interactions with the ELs, we engineered the EL1 and EL2 loops onto a soluble protein scaffold, mapping specific ligand and loop interactions using nuclear magnetic resonance spectroscopy. Key EL residues were subsequently mutated in RXFP1, and changes in function and relaxin binding were assessed alongside the RXFP1 agonist ML290 to monitor the functional integrity of the TM domain of these mutant receptors. The outcomes of this work make an important contribution to understanding the mechanism of RXFP1 activation and will aid future development of small molecule RXFP1 agonists/antagonists.
Keywords: G Protein-coupled Receptor (GPCR); Nuclear Magnetic Resonance (NMR); Peptide Hormone; Protein Engineering; RXFP1; Receptor Structure-Function; Relaxin; Serelaxin.
© 2014 by The American Society for Biochemistry and Molecular Biology, Inc.
Figures
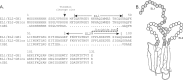
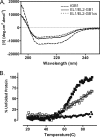
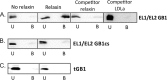
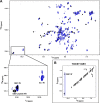
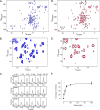
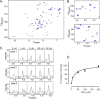
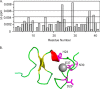
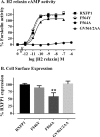
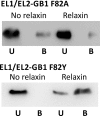
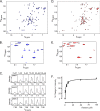
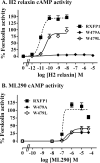
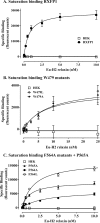
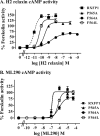
Similar articles
-
The complex binding mode of the peptide hormone H2 relaxin to its receptor RXFP1.Nat Commun. 2016 Apr 18;7:11344. doi: 10.1038/ncomms11344. Nat Commun. 2016. PMID: 27088579 Free PMC article.
-
The relaxin receptor (RXFP1) utilizes hydrophobic moieties on a signaling surface of its N-terminal low density lipoprotein class A module to mediate receptor activation.J Biol Chem. 2013 Sep 27;288(39):28138-51. doi: 10.1074/jbc.M113.499640. Epub 2013 Aug 7. J Biol Chem. 2013. PMID: 23926099 Free PMC article.
-
The NMR solution structure of the relaxin (RXFP1) receptor lipoprotein receptor class A module and identification of key residues in the N-terminal region of the module that mediate receptor activation.J Biol Chem. 2007 Feb 9;282(6):4172-84. doi: 10.1074/jbc.M609526200. Epub 2006 Dec 4. J Biol Chem. 2007. PMID: 17148455
-
Relaxin family peptide receptors--former orphans reunite with their parent ligands to activate multiple signalling pathways.Br J Pharmacol. 2007 Mar;150(6):677-91. doi: 10.1038/sj.bjp.0707140. Epub 2007 Feb 12. Br J Pharmacol. 2007. PMID: 17293890 Free PMC article. Review.
-
Membrane receptors: structure and function of the relaxin family peptide receptors.Mol Cell Endocrinol. 2010 May 14;320(1-2):1-15. doi: 10.1016/j.mce.2010.02.003. Epub 2010 Feb 6. Mol Cell Endocrinol. 2010. PMID: 20138959 Review.
Cited by
-
Expression and Characterization of Relaxin Family Peptide Receptor 1 Variants.Front Pharmacol. 2022 Jan 28;12:826112. doi: 10.3389/fphar.2021.826112. eCollection 2021. Front Pharmacol. 2022. PMID: 35153771 Free PMC article.
-
In a Class of Their Own - RXFP1 and RXFP2 are Unique Members of the LGR Family.Front Endocrinol (Lausanne). 2015 Sep 7;6:137. doi: 10.3389/fendo.2015.00137. eCollection 2015. Front Endocrinol (Lausanne). 2015. PMID: 26441827 Free PMC article. Review.
-
The relaxin family peptide receptor 1 (RXFP1): An emerging player in human health and disease.Mol Genet Genomic Med. 2020 Apr;8(4):e1194. doi: 10.1002/mgg3.1194. Epub 2020 Feb 26. Mol Genet Genomic Med. 2020. PMID: 32100955 Free PMC article. Review.
-
The complex binding mode of the peptide hormone H2 relaxin to its receptor RXFP1.Nat Commun. 2016 Apr 18;7:11344. doi: 10.1038/ncomms11344. Nat Commun. 2016. PMID: 27088579 Free PMC article.
-
Multi-Component Mechanism of H2 Relaxin Binding to RXFP1 through NanoBRET Kinetic Analysis.iScience. 2019 Jan 25;11:93-113. doi: 10.1016/j.isci.2018.12.004. Epub 2018 Dec 10. iScience. 2019. PMID: 30594862 Free PMC article.
References
-
- Bathgate R. A., Halls M. L., van der Westhuizen E. T., Callander G. E., Kocan M., Summers R. J. (2013) Relaxin family peptides and their receptors. Physiol. Rev. 93, 405–480 - PubMed
-
- Teerlink J. R., Cotter G., Davison B. A., Felker G. M., Filippatos G., Greenberg B. H., Ponikowski P., Unemori E., Voors A. A., Adams K. F., Jr., Dorobantu M. I., Grinfeld L. R., Jondeau G., Marmor A., Masip J., Pang P. S., Werdan K., Teichman S. L., Trapani A., Bush C. A., Saini R., Schumacher C., Severin T. M., Metra M. (2013) Serelaxin, recombinant human relaxin-2, for treatment of acute heart failure (RELAX-AHF): a randomised, placebo-controlled trial. Lancet 381, 29–39 - PubMed
-
- Hsu S. Y., Kudo M., Chen T., Nakabayashi K., Bhalla A., van der Spek P. J., van Duin M., Hsueh A. J. (2000) The three subfamilies of leucine-rich repeat-containing G protein-coupled receptors (LGR): identification of LGR6 and LGR7 and the signaling mechanism for LGR7. Mol. Endocrinol. 14, 1257–1271 - PubMed
-
- Büllesbach E. E., Schwabe C. (2000) The relaxin receptor-binding site geometry suggests a novel gripping mode of interaction. J. Biol. Chem. 275, 35276–35280 - PubMed
Publication types
MeSH terms
Substances
LinkOut - more resources
Full Text Sources
Other Literature Sources