Microbial sensing by Toll-like receptors and intracellular nucleic acid sensors
- PMID: 25301932
- PMCID: PMC4292165
- DOI: 10.1101/cshperspect.a016246
Microbial sensing by Toll-like receptors and intracellular nucleic acid sensors
Abstract
Recognition of an invading pathogen is critical to elicit protective responses. Certain microbial structures and molecules, which are crucial for their survival and virulence, are recognized by different families of evolutionarily conserved pattern recognition receptors (PRRs). This recognition initiates a signaling cascade that leads to the transcription of inflammatory cytokines and chemokines to eliminate pathogens and attract immune cells, thereby perpetuating further adaptive immune responses. Considerable research on the molecular mechanisms underlying host-pathogen interactions has resulted in the discovery of multifarious PRRs. In this review, we discuss the recent developments in microbial recognition by Toll-like receptors (TLRs) and intracellular nucleic acid sensors and the signaling pathways initiated by them.
Copyright © 2015 Cold Spring Harbor Laboratory Press; all rights reserved.
Figures
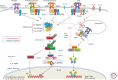
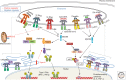
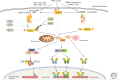
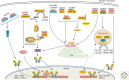
Similar articles
-
Pathogen recognition by the innate immune system.Int Rev Immunol. 2011 Feb;30(1):16-34. doi: 10.3109/08830185.2010.529976. Int Rev Immunol. 2011. PMID: 21235323 Review.
-
The role of pattern-recognition receptors in innate immunity: update on Toll-like receptors.Nat Immunol. 2010 May;11(5):373-84. doi: 10.1038/ni.1863. Epub 2010 Apr 20. Nat Immunol. 2010. PMID: 20404851 Review.
-
Toll-like receptors and innate immunity.Biochem Biophys Res Commun. 2009 Oct 30;388(4):621-5. doi: 10.1016/j.bbrc.2009.08.062. Epub 2009 Aug 15. Biochem Biophys Res Commun. 2009. PMID: 19686699 Review.
-
Microbial Nucleic Acid Sensing in Oral and Systemic Diseases.J Dent Res. 2016 Jan;95(1):17-25. doi: 10.1177/0022034515609062. Epub 2015 Oct 5. J Dent Res. 2016. PMID: 26438211 Free PMC article. Review.
-
Recognition of bacterial infection by innate immune sensors.Crit Rev Microbiol. 2013 Aug;39(3):229-46. doi: 10.3109/1040841X.2012.706249. Epub 2012 Aug 6. Crit Rev Microbiol. 2013. PMID: 22866947 Review.
Cited by
-
EGFR-mediated tyrosine phosphorylation of STING determines its trafficking route and cellular innate immunity functions.EMBO J. 2020 Nov 16;39(22):e104106. doi: 10.15252/embj.2019104106. Epub 2020 Sep 14. EMBO J. 2020. PMID: 32926474 Free PMC article.
-
Neutrophils Contribute to the Protection Conferred by ArtinM against Intracellular Pathogens: A Study on Leishmania major.PLoS Negl Trop Dis. 2016 Apr 8;10(4):e0004609. doi: 10.1371/journal.pntd.0004609. eCollection 2016 Apr. PLoS Negl Trop Dis. 2016. PMID: 27058234 Free PMC article.
-
Synergistic Targeting of Innate Receptors TLR7 and NOD2 for Therapeutic Intervention in Multiple Sclerosis.Int J Mol Sci. 2024 Jul 7;25(13):7462. doi: 10.3390/ijms25137462. Int J Mol Sci. 2024. PMID: 39000569 Free PMC article.
-
The Microbiota-Gut-Brain Axis and Alzheimer Disease. From Dysbiosis to Neurodegeneration: Focus on the Central Nervous System Glial Cells.J Clin Med. 2021 May 27;10(11):2358. doi: 10.3390/jcm10112358. J Clin Med. 2021. PMID: 34072107 Free PMC article. Review.
-
Extracellular HSPs: The Complicated Roles of Extracellular HSPs in Immunity.Front Immunol. 2016 Apr 25;7:159. doi: 10.3389/fimmu.2016.00159. eCollection 2016. Front Immunol. 2016. PMID: 27199984 Free PMC article. Review.
References
Publication types
MeSH terms
Substances
LinkOut - more resources
Full Text Sources
Other Literature Sources