Acute mTOR inhibition induces insulin resistance and alters substrate utilization in vivo
- PMID: 25161886
- PMCID: PMC4142396
- DOI: 10.1016/j.molmet.2014.06.004
Acute mTOR inhibition induces insulin resistance and alters substrate utilization in vivo
Abstract
The effect of acute inhibition of both mTORC1 and mTORC2 on metabolism is unknown. A single injection of the mTOR kinase inhibitor, AZD8055, induced a transient, yet marked increase in fat oxidation and insulin resistance in mice, whereas the mTORC1 inhibitor rapamycin had no effect. AZD8055, but not rapamycin reduced insulin-stimulated glucose uptake into incubated muscles, despite normal GLUT4 translocation in muscle cells. AZD8055 inhibited glycolysis in MEF cells. Abrogation of mTORC2 activity by SIN1 deletion impaired glycolysis and AZD8055 had no effect in SIN1 KO MEFs. Re-expression of wildtype SIN1 rescued glycolysis. Glucose intolerance following AZD8055 administration was absent in mice lacking the mTORC2 subunit Rictor in muscle, and in vivo glucose uptake into Rictor-deficient muscle was reduced despite normal Akt activity. Taken together, acute mTOR inhibition is detrimental to glucose homeostasis in part by blocking muscle mTORC2, indicating its importance in muscle metabolism in vivo.
Keywords: Glucose uptake; Glycolysis; Metabolism; Rictor; Skeletal muscle; mTORC2.
Figures
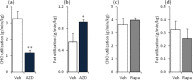
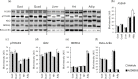
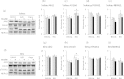
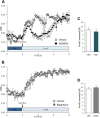
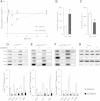
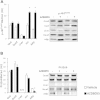
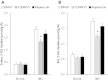
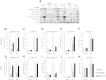
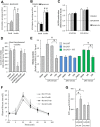
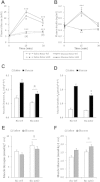
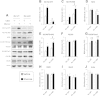
Similar articles
-
Rapamycin and mTORC2 inhibition synergistically reduce contraction-stimulated muscle protein synthesis.J Physiol. 2020 Dec;598(23):5453-5466. doi: 10.1113/JP280528. Epub 2020 Sep 23. J Physiol. 2020. PMID: 32893874
-
Muscle-specific deletion of rictor impairs insulin-stimulated glucose transport and enhances Basal glycogen synthase activity.Mol Cell Biol. 2008 Jan;28(1):61-70. doi: 10.1128/MCB.01405-07. Epub 2007 Oct 29. Mol Cell Biol. 2008. PMID: 17967879 Free PMC article.
-
Fat cell-specific ablation of rictor in mice impairs insulin-regulated fat cell and whole-body glucose and lipid metabolism.Diabetes. 2010 Jun;59(6):1397-406. doi: 10.2337/db09-1061. Epub 2010 Mar 23. Diabetes. 2010. PMID: 20332342 Free PMC article.
-
Diverse signaling mechanisms of mTOR complexes: mTORC1 and mTORC2 in forming a formidable relationship.Adv Biol Regul. 2019 May;72:51-62. doi: 10.1016/j.jbior.2019.03.003. Epub 2019 Apr 11. Adv Biol Regul. 2019. PMID: 31010692 Review.
-
Adrenoceptors promote glucose uptake into adipocytes and muscle by an insulin-independent signaling pathway involving mechanistic target of rapamycin complex 2.Pharmacol Res. 2017 Feb;116:87-92. doi: 10.1016/j.phrs.2016.12.022. Epub 2016 Dec 23. Pharmacol Res. 2017. PMID: 28025104 Review.
Cited by
-
Reply from Lykke Sylow, Lisbeth L. V. Møller, Maximilian Kleinert, Erik A. Richter and Thomas E. Jensen.J Physiol. 2015 May 1;593(9):2239-40. doi: 10.1113/JP270414. J Physiol. 2015. PMID: 25931408 Free PMC article. No abstract available.
-
Associations between Diabetes Mellitus and Selected Cancers.Int J Mol Sci. 2024 Jul 8;25(13):7476. doi: 10.3390/ijms25137476. Int J Mol Sci. 2024. PMID: 39000583 Free PMC article. Review.
-
Age-Related Neurodegeneration Prevention Through mTOR Inhibition: Potential Mechanisms and Remaining Questions.Curr Top Med Chem. 2015;15(21):2139-51. doi: 10.2174/1568026615666150610125856. Curr Top Med Chem. 2015. PMID: 26059360 Free PMC article. Review.
-
Simvastatin Impairs Glucose Homeostasis in Mice Depending on PGC-1α Skeletal Muscle Expression.Biomedicines. 2020 Sep 15;8(9):351. doi: 10.3390/biomedicines8090351. Biomedicines. 2020. PMID: 32942550 Free PMC article.
-
Glucometabolic consequences of acute and prolonged inhibition of fatty acid oxidation.J Lipid Res. 2020 Jan;61(1):10-19. doi: 10.1194/jlr.RA119000177. Epub 2019 Nov 12. J Lipid Res. 2020. PMID: 31719103 Free PMC article.
References
-
- Hagiwara A., Cornu M., Cybulski N., Polak P., Betz C., Trapani F. Hepatic MTORC2 activates glycolysis and lipogenesis through Akt, glucokinase, and SREBP1c. Cell Metabolism. 2012;15:725–738. - PubMed
LinkOut - more resources
Full Text Sources
Other Literature Sources
Research Materials
Miscellaneous