Membrane transporters for the special amino acid glutamine: structure/function relationships and relevance to human health
- PMID: 25157349
- PMCID: PMC4127817
- DOI: 10.3389/fchem.2014.00061
Membrane transporters for the special amino acid glutamine: structure/function relationships and relevance to human health
Abstract
Glutamine together with glucose is essential for body's homeostasis. It is the most abundant amino acid and is involved in many biosynthetic, regulatory and energy production processes. Several membrane transporters which differ in transport modes, ensure glutamine homeostasis by coordinating its absorption, reabsorption and delivery to tissues. These transporters belong to different protein families, are redundant and ubiquitous. Their classification, originally based on functional properties, has recently been associated with the SLC nomenclature. Function of glutamine transporters is studied in cells over-expressing the transporters or, more recently in proteoliposomes harboring the proteins extracted from animal tissues or over-expressed in microorganisms. The role of the glutamine transporters is linked to their transport modes and coupling with Na(+) and H(+). Most transporters share specificity for other neutral or cationic amino acids. Na(+)-dependent co-transporters efficiently accumulate glutamine while antiporters regulate the pools of glutamine and other amino acids. The most acknowledged glutamine transporters belong to the SLC1, 6, 7, and 38 families. The members involved in the homeostasis are the co-transporters B0AT1 and the SNAT members 1, 2, 3, 5, and 7; the antiporters ASCT2, LAT1 and 2. The last two are associated to the ancillary CD98 protein. Some information on regulation of the glutamine transporters exist, which, however, need to be deepened. No information at all is available on structures, besides some homology models obtained using similar bacterial transporters as templates. Some models of rat and human glutamine transporters highlight very similar structures between the orthologs. Moreover the presence of glycosylation and/or phosphorylation sites located at the extracellular or intracellular faces has been predicted. ASCT2 and LAT1 are over-expressed in several cancers, thus representing potential targets for pharmacological intervention.
Keywords: amino acids; cancer; glutamine; homology models; membrane; nutrients; transporters.
Figures
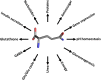
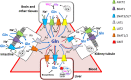
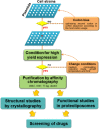
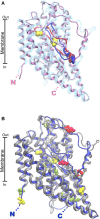
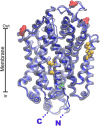
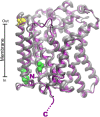
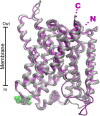
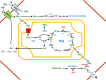
Similar articles
-
The glutamine transporter ASCT2 (SLC1A5) promotes tumor growth independently of the amino acid transporter LAT1 (SLC7A5).J Biol Chem. 2018 Feb 23;293(8):2877-2887. doi: 10.1074/jbc.RA117.001342. Epub 2018 Jan 11. J Biol Chem. 2018. PMID: 29326164 Free PMC article.
-
High-affinity glutamate transporter GLAST/EAAT1 regulates cell surface expression of glutamine/neutral amino acid transporter ASCT2 in human fetal astrocytes.Neurochem Int. 2006 May-Jun;48(6-7):611-5. doi: 10.1016/j.neuint.2005.12.033. Epub 2006 Mar 3. Neurochem Int. 2006. PMID: 16516348
-
Glutamine Transport and Mitochondrial Metabolism in Cancer Cell Growth.Front Oncol. 2017 Dec 11;7:306. doi: 10.3389/fonc.2017.00306. eCollection 2017. Front Oncol. 2017. PMID: 29376023 Free PMC article. Review.
-
The astroglial ASCT2 amino acid transporter as a mediator of glutamine efflux.J Neurochem. 1999 Nov;73(5):2184-94. J Neurochem. 1999. PMID: 10537079
-
Recent molecular advances in mammalian glutamine transport.J Nutr. 2001 Sep;131(9 Suppl):2475S-85S; discussion 2486S-7S. doi: 10.1093/jn/131.9.2475S. J Nutr. 2001. PMID: 11533296 Review.
Cited by
-
Cu-Catalyzed Azide-Alkyne-Thiol Reaction Forms Ubiquitous Background in Chemical Proteomic Studies.J Am Chem Soc. 2024 Jan 24;146(3):2151-2159. doi: 10.1021/jacs.3c11780. Epub 2024 Jan 12. J Am Chem Soc. 2024. PMID: 38214237 Free PMC article.
-
Nutrients and the microenvironment to feed a T cell army.Semin Immunol. 2016 Oct;28(5):505-513. doi: 10.1016/j.smim.2016.09.003. Epub 2016 Oct 3. Semin Immunol. 2016. PMID: 27712958 Free PMC article. Review.
-
Evidence of adaptation of maternofetal transport of glutamine relative to placental size in normal mice, and in those with fetal growth restriction.J Physiol. 2019 Oct;597(19):4975-4990. doi: 10.1113/JP278226. Epub 2019 Aug 27. J Physiol. 2019. PMID: 31400764 Free PMC article.
-
Cancer as a Metabolic Disorder.Int J Mol Sci. 2022 Jan 21;23(3):1155. doi: 10.3390/ijms23031155. Int J Mol Sci. 2022. PMID: 35163079 Free PMC article. Review.
-
Targeting Pulmonary Fibrosis by SLC1A5-Dependent Glutamine Transport Blockade.Am J Respir Cell Mol Biol. 2023 Oct;69(4):441-455. doi: 10.1165/rcmb.2022-0339OC. Am J Respir Cell Mol Biol. 2023. PMID: 37459644 Free PMC article.
References
Publication types
LinkOut - more resources
Full Text Sources
Other Literature Sources
Research Materials