TPC1 has two variant isoforms, and their removal has different effects on endo-lysosomal functions compared to loss of TPC2
- PMID: 25135478
- PMCID: PMC4386455
- DOI: 10.1128/MCB.00113-14
TPC1 has two variant isoforms, and their removal has different effects on endo-lysosomal functions compared to loss of TPC2
Abstract
Organelle ion homeostasis within the endo-lysosomal system is critical for physiological functions. Two-pore channels (TPCs) are cation channels that reside in endo-lysosomal organelles, and overexpression results in endo-lysosomal trafficking defects. However, the impact of a lack of TPC expression on endo-lysosomal trafficking is unknown. Here, we characterize Tpcn1 expression in two transgenic mouse lines (Tpcn1(XG716) and Tpcn1(T159)) and show expression of a novel evolutionarily conserved Tpcn1B transcript from an alternative promoter, raising important questions regarding the status of Tpcn1 expression in mice recently described to be Tpcn1 knockouts. We show that the transgenic Tpcn1(T159) line lacks expression of both Tpcn1 isoforms in all tissues analyzed. Using mouse embryonic fibroblasts (MEFs) from Tpcn1(-/-) and Tpcn2(-/-) animals, we show that a lack of Tpcn1 or Tpcn2 expression has no significant impact on resting endo-lysosomal pH or morphology. However, differential effects in endo-lysosomal function were observed upon the loss of Tpcn1 or Tpcn2 expression; thus, while Tpcn1(-/-) MEFs have impaired trafficking of cholera toxin from the plasma membrane to the Golgi apparatus, Tpcn2(-/-) MEFs show slower kinetics of ligand-induced platelet-derived growth factor receptor β (PDGFRβ) degradation, which is dependent on trafficking to lysosomes. Our findings indicate that TPC1 and TPC2 have important but distinct roles in the endo-lysosomal pathway.
Copyright © 2014 Ruas et al.
Figures
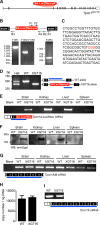
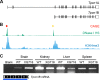
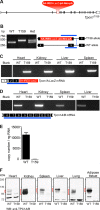
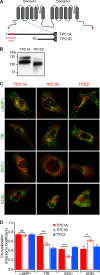
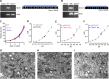
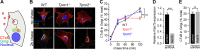
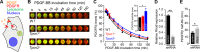
Comment in
-
TPC1 Knockout Knocks Out TPC1.Mol Cell Biol. 2015 May;35(10):1882-3. doi: 10.1128/MCB.00020-15. Mol Cell Biol. 2015. PMID: 25900974 Free PMC article. No abstract available.
-
Reply to "TPC1 Knockout Knocks Out TPC1".Mol Cell Biol. 2015 May;35(10):1884. doi: 10.1128/MCB.00083-15. Mol Cell Biol. 2015. PMID: 25900975 Free PMC article. No abstract available.
Similar articles
-
Expression of Ca²⁺-permeable two-pore channels rescues NAADP signalling in TPC-deficient cells.EMBO J. 2015 Jul 2;34(13):1743-58. doi: 10.15252/embj.201490009. Epub 2015 Apr 14. EMBO J. 2015. PMID: 25872774 Free PMC article.
-
Agonist-specific voltage-dependent gating of lysosomal two-pore Na+ channels.Elife. 2019 Dec 11;8:e51423. doi: 10.7554/eLife.51423. Elife. 2019. PMID: 31825310 Free PMC article.
-
Activation of endo-lysosomal two-pore channels by NAADP and PI(3,5)P2. Five things to know.Cell Calcium. 2022 May;103:102543. doi: 10.1016/j.ceca.2022.102543. Epub 2022 Jan 25. Cell Calcium. 2022. PMID: 35123238 Free PMC article. Review.
-
Endolysosomal two-pore channels regulate autophagy in cardiomyocytes.J Physiol. 2016 Jun 1;594(11):3061-77. doi: 10.1113/JP271332. Epub 2016 Feb 4. J Physiol. 2016. PMID: 26757341 Free PMC article.
-
From mucolipidosis type IV to Ebola: TRPML and two-pore channels at the crossroads of endo-lysosomal trafficking and disease.Cell Calcium. 2017 Nov;67:148-155. doi: 10.1016/j.ceca.2017.04.003. Epub 2017 Apr 23. Cell Calcium. 2017. PMID: 28457591 Review.
Cited by
-
Mining of Ebola virus entry inhibitors identifies approved drugs as two-pore channel pore blockers.Biochim Biophys Acta Mol Cell Res. 2019 Jul;1866(7):1151-1161. doi: 10.1016/j.bbamcr.2018.10.022. Epub 2018 Nov 5. Biochim Biophys Acta Mol Cell Res. 2019. PMID: 30408544 Free PMC article.
-
The Relevance of Aquaporins for the Physiology, Pathology, and Aging of the Female Reproductive System in Mammals.Cells. 2020 Dec 1;9(12):2570. doi: 10.3390/cells9122570. Cells. 2020. PMID: 33271827 Free PMC article. Review.
-
Two-pore channels (TPCs): Novel voltage-gated ion channels with pleiotropic functions.Channels (Austin). 2017 Jan 2;11(1):20-33. doi: 10.1080/19336950.2016.1213929. Epub 2016 Jul 20. Channels (Austin). 2017. PMID: 27440385 Free PMC article. Review.
-
Reply to "TPC1 Knockout Knocks Out TPC1".Mol Cell Biol. 2015 May;35(10):1884. doi: 10.1128/MCB.00083-15. Mol Cell Biol. 2015. PMID: 25900975 Free PMC article. No abstract available.
-
NAADP-dependent Ca2+ signaling regulates Middle East respiratory syndrome-coronavirus pseudovirus translocation through the endolysosomal system.Cell Calcium. 2018 Nov;75:30-41. doi: 10.1016/j.ceca.2018.08.003. Epub 2018 Aug 9. Cell Calcium. 2018. PMID: 30121440 Free PMC article.
References
Publication types
MeSH terms
Substances
Grants and funding
LinkOut - more resources
Full Text Sources
Other Literature Sources
Molecular Biology Databases
Research Materials
Miscellaneous