RNA G-quadruplexes cause eIF4A-dependent oncogene translation in cancer
- PMID: 25079319
- PMCID: PMC4492470
- DOI: 10.1038/nature13485
RNA G-quadruplexes cause eIF4A-dependent oncogene translation in cancer
Abstract
The translational control of oncoprotein expression is implicated in many cancers. Here we report an eIF4A RNA helicase-dependent mechanism of translational control that contributes to oncogenesis and underlies the anticancer effects of silvestrol and related compounds. For example, eIF4A promotes T-cell acute lymphoblastic leukaemia development in vivo and is required for leukaemia maintenance. Accordingly, inhibition of eIF4A with silvestrol has powerful therapeutic effects against murine and human leukaemic cells in vitro and in vivo. We use transcriptome-scale ribosome footprinting to identify the hallmarks of eIF4A-dependent transcripts. These include 5' untranslated region (UTR) sequences such as the 12-nucleotide guanine quartet (CGG)4 motif that can form RNA G-quadruplex structures. Notably, among the most eIF4A-dependent and silvestrol-sensitive transcripts are a number of oncogenes, superenhancer-associated transcription factors, and epigenetic regulators. Hence, the 5' UTRs of select cancer genes harbour a targetable requirement for the eIF4A RNA helicase.
Conflict of interest statement
The authors declare no competing financial interests.
Figures
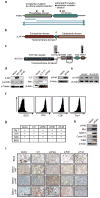
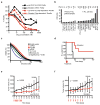
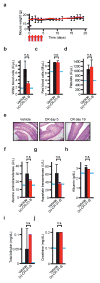
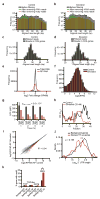
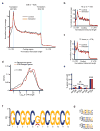
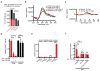
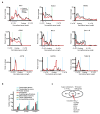
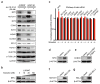
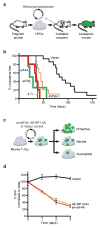
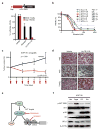
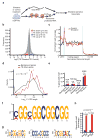
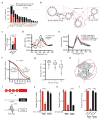
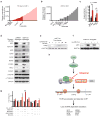
Similar articles
-
Mechanistic differences in eukaryotic initiation factor requirements for eIF4GI-driven cap-independent translation of structured mRNAs.J Biol Chem. 2024 Nov;300(11):107866. doi: 10.1016/j.jbc.2024.107866. Epub 2024 Oct 9. J Biol Chem. 2024. PMID: 39384039 Free PMC article.
-
Vibrio cholerae CsrA controls ToxR levels by increasing the stability and translation of toxR mRNA.mBio. 2024 Dec 11;15(12):e0285324. doi: 10.1128/mbio.02853-24. Epub 2024 Nov 18. mBio. 2024. PMID: 39555915 Free PMC article.
-
The Emerging Roles of Multimolecular G-Quadruplexes in Transcriptional Regulation and Chromatin Organization.Acc Chem Res. 2024 Dec 3;57(23):3397-3406. doi: 10.1021/acs.accounts.4c00574. Epub 2024 Nov 18. Acc Chem Res. 2024. PMID: 39555660 Free PMC article.
-
Searching for IRES.RNA. 2006 Oct;12(10):1755-85. doi: 10.1261/rna.157806. Epub 2006 Sep 6. RNA. 2006. PMID: 16957278 Free PMC article. Review.
-
Depressing time: Waiting, melancholia, and the psychoanalytic practice of care.In: Kirtsoglou E, Simpson B, editors. The Time of Anthropology: Studies of Contemporary Chronopolitics. Abingdon: Routledge; 2020. Chapter 5. In: Kirtsoglou E, Simpson B, editors. The Time of Anthropology: Studies of Contemporary Chronopolitics. Abingdon: Routledge; 2020. Chapter 5. PMID: 36137063 Free Books & Documents. Review.
Cited by
-
Irregular G-quadruplexes Found in the Untranslated Regions of Human mRNAs Influence Translation.J Biol Chem. 2016 Oct 7;291(41):21751-21760. doi: 10.1074/jbc.M116.744839. Epub 2016 Aug 24. J Biol Chem. 2016. PMID: 27557661 Free PMC article.
-
Inhibition of translation initiation factor eIF4a inactivates heat shock factor 1 (HSF1) and exerts anti-leukemia activity in AML.Leukemia. 2021 Sep;35(9):2469-2481. doi: 10.1038/s41375-021-01308-z. Epub 2021 Jun 14. Leukemia. 2021. PMID: 34127794 Free PMC article. Review.
-
Fine-tuning of macrophage activation using synthetic rocaglate derivatives.Sci Rep. 2016 Apr 18;6:24409. doi: 10.1038/srep24409. Sci Rep. 2016. PMID: 27086720 Free PMC article.
-
Translational control by 5'-untranslated regions of eukaryotic mRNAs.Science. 2016 Jun 17;352(6292):1413-6. doi: 10.1126/science.aad9868. Science. 2016. PMID: 27313038 Free PMC article. Review.
-
New frontiers in translational control of the cancer genome.Nat Rev Cancer. 2016 Apr 26;16(5):288-304. doi: 10.1038/nrc.2016.27. Nat Rev Cancer. 2016. PMID: 27112207 Free PMC article. Review.
References
-
- Wendel HG, et al. Survival signalling by Akt and eIF4E in oncogenesis and cancer therapy. Nature. 2004;428:332–337. - PubMed
-
- Mamane Y, Petroulakis E, LeBacquer O, Sonenberg N. mTOR, translation initiation and cancer. Oncogene. 2006;25:6416–6422. - PubMed
-
- Rajasekhar VK, et al. Oncogenic Ras and Akt signaling contribute to glioblastoma formation by differential recruitment of existing mRNAs to polysomes. Molecular cell. 2003;12:889–901. - PubMed
-
- Lazaris-Karatzas A, Montine KS, Sonenberg N. Malignant transformation by a eukaryotic initiation factor subunit that binds to mRNA 5′ cap. Nature. 1990;345:544–547. - PubMed
Publication types
MeSH terms
Substances
Associated data
- Actions
Grants and funding
LinkOut - more resources
Full Text Sources
Other Literature Sources
Molecular Biology Databases
Research Materials
Miscellaneous