A myeloperoxidase-containing complex regulates neutrophil elastase release and actin dynamics during NETosis
- PMID: 25066128
- PMCID: PMC4471680
- DOI: 10.1016/j.celrep.2014.06.044
A myeloperoxidase-containing complex regulates neutrophil elastase release and actin dynamics during NETosis
Abstract
Neutrophils contain granules loaded with antimicrobial proteins and are regarded as impermeable organelles that deliver cargo via membrane fusion. However, during the formation of neutrophil extracellular traps (NETs), neutrophil elastase (NE) translocates from the granules to the nucleus via an unknown mechanism that does not involve membrane fusion and requires reactive oxygen species (ROS). Here, we show that the ROS triggers the dissociation of NE from a membrane-associated complex into the cytosol and activates its proteolytic activity in a myeloperoxidase (MPO)-dependent manner. In the cytosol, NE first binds and degrades F-actin to arrest actin dynamics and subsequently translocates to the nucleus. The complex is an example of an oxidative signaling scaffold that enables ROS and antimicrobial proteins to regulate neutrophil responses. Furthermore, granules contain protein machinery that transports and delivers cargo across membranes independently of membrane fusion.
Copyright © 2014 The Authors. Published by Elsevier Inc. All rights reserved.
Figures
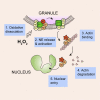
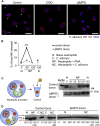
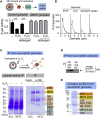
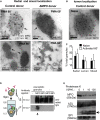
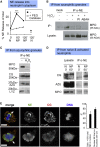
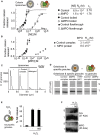
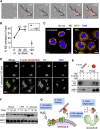
Similar articles
-
Classical ROS-dependent and early/rapid ROS-independent release of Neutrophil Extracellular Traps triggered by Leishmania parasites.Sci Rep. 2015 Dec 17;5:18302. doi: 10.1038/srep18302. Sci Rep. 2015. PMID: 26673780 Free PMC article.
-
Entamoeba histolytica Trophozoites Induce a Rapid Non-classical NETosis Mechanism Independent of NOX2-Derived Reactive Oxygen Species and PAD4 Activity.Front Cell Infect Microbiol. 2018 Jun 5;8:184. doi: 10.3389/fcimb.2018.00184. eCollection 2018. Front Cell Infect Microbiol. 2018. PMID: 29922599 Free PMC article.
-
Neutrophil elastase and myeloperoxidase regulate the formation of neutrophil extracellular traps.J Cell Biol. 2010 Nov 1;191(3):677-91. doi: 10.1083/jcb.201006052. Epub 2010 Oct 25. J Cell Biol. 2010. PMID: 20974816 Free PMC article.
-
[Progress in mechanism of formation of neutrophil extracellular traps: Review].Xi Bao Yu Fen Zi Mian Yi Xue Za Zhi. 2020 Jun;36(6):561-564. Xi Bao Yu Fen Zi Mian Yi Xue Za Zhi. 2020. PMID: 32696748 Review. Chinese.
-
Neutrophil Elastase and Neutrophil Extracellular Traps in the Tumor Microenvironment.Adv Exp Med Biol. 2020;1263:13-23. doi: 10.1007/978-3-030-44518-8_2. Adv Exp Med Biol. 2020. PMID: 32588320 Review.
Cited by
-
PAD4 and Its Inhibitors in Cancer Progression and Prognosis.Pharmaceutics. 2022 Nov 8;14(11):2414. doi: 10.3390/pharmaceutics14112414. Pharmaceutics. 2022. PMID: 36365233 Free PMC article. Review.
-
A Higher Abundance of O-Linked Glycans Confers a Selective Advantage to High Fertile Buffalo Spermatozoa for Immune-Evasion From Neutrophils.Front Immunol. 2020 Aug 28;11:1928. doi: 10.3389/fimmu.2020.01928. eCollection 2020. Front Immunol. 2020. PMID: 32983120 Free PMC article.
-
The molecular mechanisms of MLKL-dependent and MLKL-independent necrosis.J Mol Cell Biol. 2021 Apr 10;13(1):3-14. doi: 10.1093/jmcb/mjaa055. J Mol Cell Biol. 2021. PMID: 33064829 Free PMC article. Review.
-
Proteins associated with neutrophil degranulation are upregulated in nasopharyngeal swabs from SARS-CoV-2 patients.PLoS One. 2020 Oct 20;15(10):e0240012. doi: 10.1371/journal.pone.0240012. eCollection 2020. PLoS One. 2020. PMID: 33079950 Free PMC article.
-
PRL2 regulates neutrophil extracellular trap formation which contributes to severe malaria and acute lung injury.Nat Commun. 2024 Jan 29;15(1):881. doi: 10.1038/s41467-024-45210-5. Nat Commun. 2024. PMID: 38286811 Free PMC article.
References
-
- Aga E., Katschinski D.M., van Zandbergen G., Laufs H., Hansen B., Müller K., Solbach W., Laskay T. Inhibition of the spontaneous apoptosis of neutrophil granulocytes by the intracellular parasite Leishmania major. J. Immunol. 2002;169:898–905. - PubMed
-
- Ahrens S., Zelenay S., Sancho D., Hanč P., Kjær S., Feest C., Fletcher G., Durkin C., Postigo A., Skehel M. F-actin is an evolutionarily conserved damage-associated molecular pattern recognized by DNGR-1, a receptor for dead cells. Immunity. 2012;36:635–645. - PubMed
-
- Amulic B., Cazalet C., Hayes G.L., Metzler K.D., Zychlinsky A. Neutrophil function: from mechanisms to disease. Annu. Rev. Immunol. 2012;30:459–489. - PubMed
-
- Anderluh G., Lakey J. Proteins: membrane binding and pore formation. Preface. Adv. Exp. Med. Biol. 2010;677:v–vi. - PubMed
-
- Borregaard N. Neutrophils, from marrow to microbes. Immunity. 2010;33:657–670. - PubMed
Publication types
MeSH terms
Substances
Grants and funding
LinkOut - more resources
Full Text Sources
Other Literature Sources
Research Materials
Miscellaneous